Advancing knowledge in mitochondria division using Dragonfly ultra-fast imaging
Sponsored by Andor, on 1 July 2020
Mitochondria are eukaryotic cellular organelles that are believed to have evolved from a prokaryotic symbiote inside a eukaryotic cell. This small organelle is of vital importance to the cells and organisms since it is the source of cellular energy. Besides their crucial role as an ATP generating unit of the cells, mitochondria have other vital cellular functions in cellular signalling, apoptosis and cell differentiation. Not surprisingly, defects in mitochondrial dynamics cause severe disorders such as neurodegenerative and cardiovascular disorders, neurometabolic diseases, cancer, obesity, among others.
Glossary
Dpr1 | A dynamin GTPase required for outer mitochondrial membrane division |
Dynamin | A crucial GTPase for clathrin-dependent endocytosis |
ER | Endoplasmatic reticulum |
ETC | Electron transport chain |
formins | Proteins implicated actin polymerisation. |
IMM | Inner mitochondrial membrane |
INF2 | Inverted Formin 2 is a unique formin that can both polymerise and depolymerise actin filaments. Mutations in INF2 cause kidney disease focal and segmental glomerulosclerosis. INF2 can be expressed as two C-terminal splice variants: CAAX and non-CAAX. |
INF2-CAAX | The INF2-CAAX isoform is tightly bound to the endoplasmic reticulum (ER) |
Ionomycin | A bacterial ionophore used in research to elevate the intracellular level of calcium (Ca2+) and as a tool to decipher Ca2+ transport across biological membranes. |
KD | Knockdown |
KO | Knock out |
latrunculin A | (LatA) – an actin sequestering molecule |
MCU | Mitochondrial Calcium Uniporter is a major inner mitochondrial membrane protein and accounts for most of mitochondrial calcium entry |
MCU-KD | MCU knockdown by RNAi. |
mitophagy | The selective degradation of mitochondria by autophagy |
OMM | Outer mitochondrial membrane |
Thapsigargin | SERCA ATPase inhibitor that promotes ER calcium leak and thereby depletes the calcium from the endoplasmatic reticulum. |
RNAi | RNA interference |
An image of mitochondria by electron microscopy shows that this organelle has a characteristic double membrane: the outer mitochondrial membrane (OMM) which is in contact with the cytoplasm and the inner mitochondrial membrane (IMM) that is thrown into folds of cristae housing the ATP generating machinery. Recent advances in microscopy have transformed the perception of mitochondria from being static and isolated structures to be dynamic organelles whose configuration varies continuously in the cell.
In cells mitochondria modify their morphology from being small individual units (during cell division) to reticular hyperfused network (during cell growth phase). Mitochondrial dynamics are the result of highly regulated and coordinated fission and fusion events [1]. Fusion is the process by which two individual mitochondrion fuse to mix their contents and become one entity; Fission is the division of mitochondria. Both fusion and fission are highly dynamic con-trolled processes, and only recently, the detailed mechanism and molecular players have begun to be uncovered. Not surprisingly, both fusion and fission when de-regulated can cause mitochondrial dis-eases. Therefore, the study of mitochondrial dynamics goes beyond the scope of basic biology and reaches out to understand the molecular basis of diseases.
Studies have shown that endoplasmatic reticulum (ER) is intricately placed at mitochondrial division sites. It is believed that ER forms a tube that contacts and wraps around mitochondria promoting the pre-constriction step [2]. The subsequent step is the assembly of the fission machinery and the oligomerisation of the dynamin family GTPase Drp1 at the outer mitochondrial membrane (OMM) [1] where its GTP hydrolysis provides a constrictive force to divide the mitochondria. Actin filaments were shown to be an essential mediator for the entire mechanism. The ER-bound inverted-formin 2 (INF2) is an actin polymerization factor whose depletion impairs both actin polymerisation at the mitochondria-ER interface and mitochondria fission resulting in elongated mitochondria [1]. Previously research conducted in the Higgs lab has shown that actin polymerisation driven by the ER-bound inverted formin 2 (INF2) promotes functional Drp1 recruitment and oligomerisation to the OMM in mammalian cells [3,4]. However, the mitochondrial division consists of the partition of both the inner and outer mitochondrial membranes (IMM and OMM). Several studies have covered the mechanism of OMM in mitochondria division, but the role and mechanism of IMM division is barely studied. Recent work done by Dr Rajarshi Chakrabarti and colleagues from Professor Henry Higgs’ lab have highlighted the signalling processes of IMM in mitochondrial division [5] (Fig. 1).
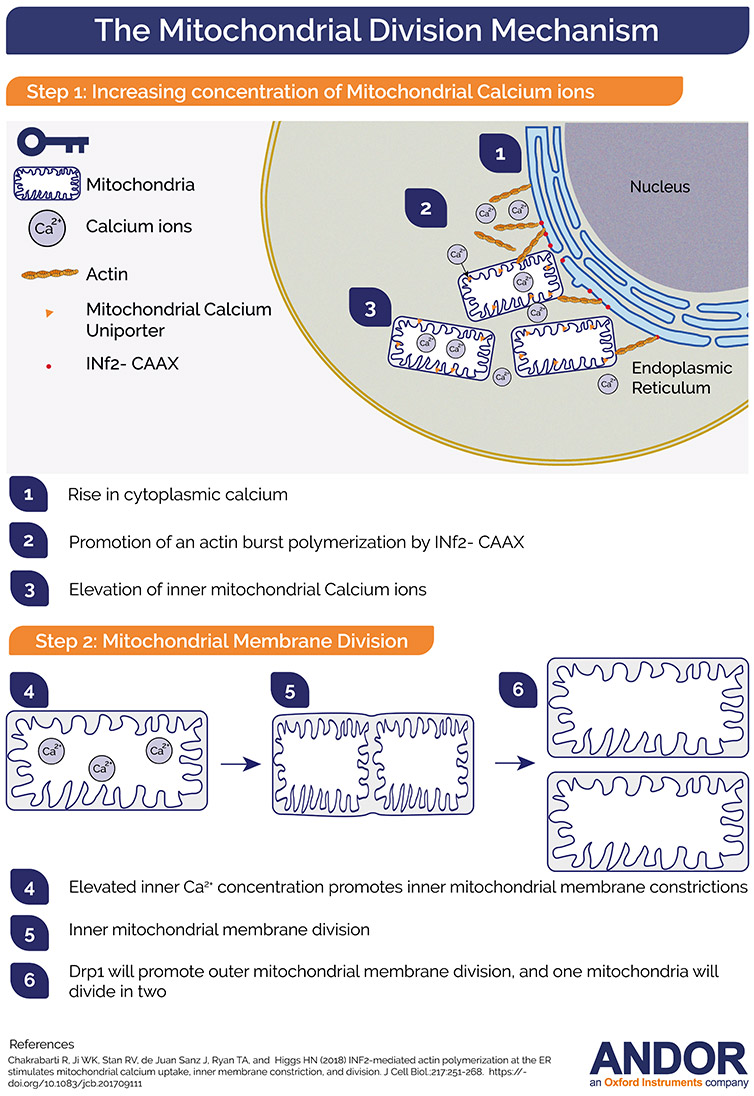
It was previously shown that an increase in cytoplasmatic Ca2+ would trigger a burst of actin polymerisation. This cytoplasmic calcium increase could be driven by different stimuli such as ionomycin and histamine. Having this in mind, the authors wanted to investigate the role of this elevated concentration of Ca2+ (either cytoplasmic or mitochondrial) in mitochondrial division. Imaging intercellular calcium concentration would require a microscope that could acquire at high speeds with extreme sensitivity. The authors have chosen Dragonfly “due to its ability to acquire high temporal resolution live imaging data”[6].
The combination of calcium probes and an ultra-fast imaging system (Dragonfly) gave the authors the tools to decipher the temporal order of calcium dynamics in the cytosol, ER and mitochondria and its relation to actin filament formation. The obtained results suggested that calcium transfer to mitochondrial matrix upon ionomycin or histamine stimulation occurs entirely from ER stores.
Similarly, and linked with the previous findings, the authors asked if the actin burst that is observed to precede the increase in mitochondrial calcium could drive this signalling. The experiment to test this hypothesis was the treatment of the cells with latrunculin A (which impaired actin polymerisation) and measure the mitochondrial matrix calcium spike upon stimulation with histamine or ionomycin. The authors concluded that the actin burst is required for mitochondrial calcium entry: when actin polymerisation was impaired mitochondrial calcium entry was strongly inhibited (Fig. 2).
INF2 is an actin polymerising protein that has an ER bound variant: INF2-CAAX. The Higgs lab had previously demonstrated that INF2-CAAX is required for mitochondrial division and for the actin burst that precedes the division [3,4]. Therefore, the authors investigated if INF2-CAAX would play a role in the mitochondrial calcium spike. The conclusion was, that INF2 is important for the stimulus induced mitochondrial calcium spike. Importantly, the ER localisation is a crucial factor. Moreover, actin polymerisation by the ER bound form of INF2 enhances ER-mitochondrial contacts, which will subsequently cause a rise in the stimulus-induced mitochondrial calcium.
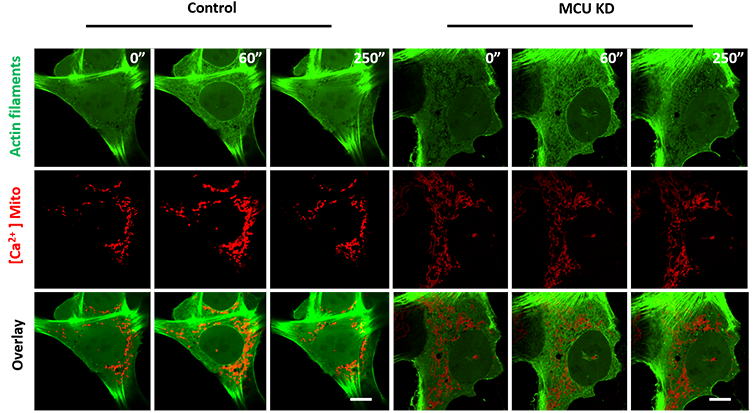
The authors observed that Ionomycin causes a 6.7-fold increase in mitochondrial constrictions. Significantly, the timing of these constrictions matched the timing of the mitochondrial calcium spikes.
Because Drp-1 is a protein required for OMM constriction, it´s role on the Ionomycin induced constrictions was investigated. It turned out that these constrictions were not driven by the OMM, or by Drp1. Therefore, the authors were on the verge of a discovery of a new mechanism in the process of mitochondrial division.
Using MCU-KD cells, the authors observed that there was a significant decrease in the number of ionomycin induced mitochondrial constrictions. Could inner mitochondrial membrane dynamics cause the mitochondrial constrictions? The answer to this question was yes. The researchers went even further and discovered a requirement for the functional electron transport chain to trigger inner mitochondrial membrane constrictions which were a priming event for complete mitochondrial division (Fig. 3).
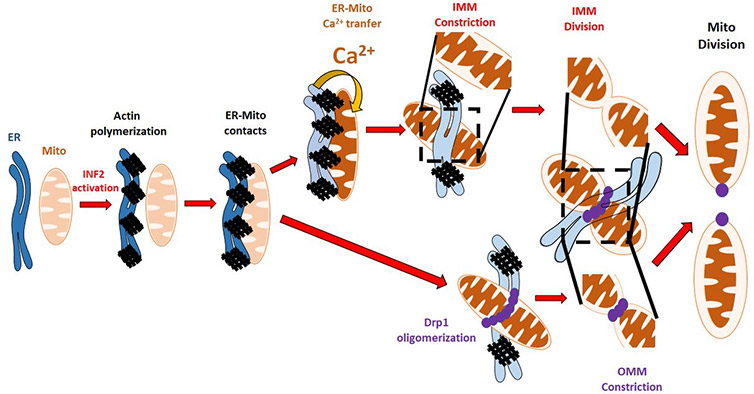
In conclusion, ultra-fast imaging and acquisition made possible with the Andor Dragonfly and Zyla 4.2 sCMOS camera allowed these scientists to elucidate the mechanism of mitochondrial division. We now know that besides the role of Drp-1 in promoting the constriction and division of the outer mitochondrial membrane, there is a newly uncovered mechanism that promotes the constrictions of the inner mitochondrial membrane, and this process is driven by intra-mitochondrial calcium signalling.