Temperature control in light microscopy – challenges and solutions
Sponsored by Interherence, on 9 September 2021
We describe common challenges that researchers face when trying to precisely control the temperature of the sample they are imaging. We aim to show that thermal control in high sensitivity microscopy is not trivial if you care about temperature directly in the field of view of the microscope (which you should). We hope to persuade you that it pays off to focus on temperature control more. You will be rewarded by reliable and reproducible data.
All processes in nature are temperature dependent. For example, an old rule of thumb in chemistry says that the rate of chemical reactions will double with every 10°C increase. Therefore, it is important to always perform your experiment at a defined temperature. Relying on “laboratory temperature” is not a good idea because that value changes a lot between summer and winter, when AC is on and off, whether there is hot air blowing from a fan of a large instrument next to your experiment, etc.
This recommendation to always control temperature in your experiments is universally valid including imaging experiments. Additionally, when dealing with biological samples, it is important to consider that the living systems evolved to function at a certain physiological temperature and this is the temperature at which they should be studied in most cases. Variation of just few °C dramatically changes the speed of enzymatic reactions, protein diffusion, cytoskeletal dynamics, membrane fluidity or might even trigger irreversible phase transitions. That is why your temperature control solution has to be more precise than that!
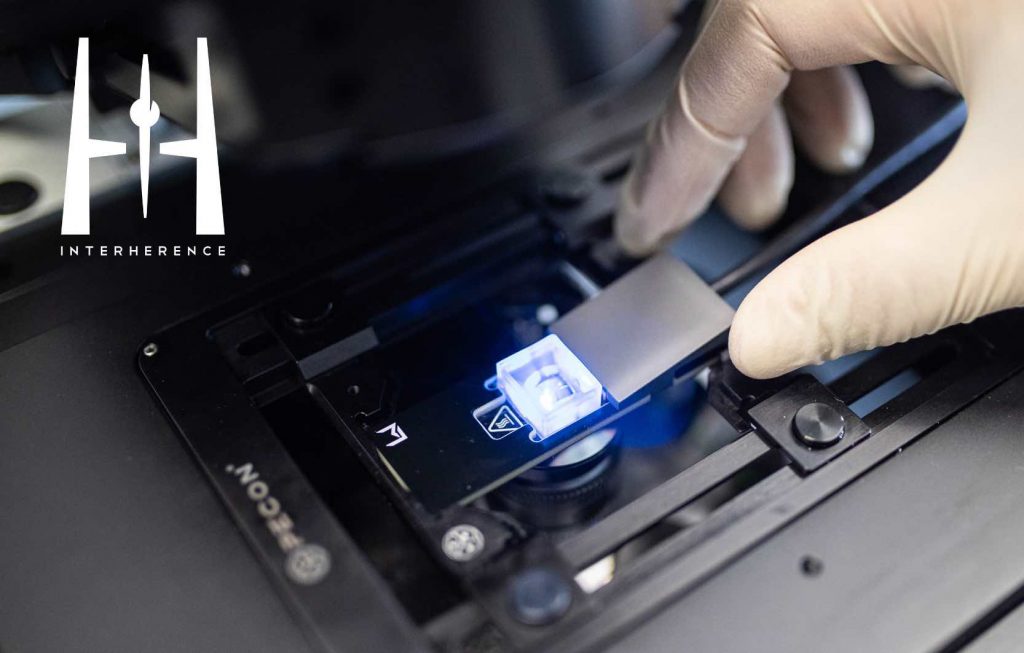
Challenging aspects of temperature control in microscopy
What are the challenges connected with temperature control in imaging experiments?
- Liquid sample evaporation – media concentration changes, condensation on colder surfaces
- Thermal drift of the sample
- Limited temperature range (45–55°C max.) and no possibility to do fast temperature ramps in common control systems
- Degradation of image quality or loss of TIRF angle at higher temperatures
- Complexity of some setups – multiple feedback loops, specific temperature calibration necessary
- Undefined temperature and a gradient across the field of view – immersion objective acting as a heat sink
The first challenge is evaporation, which causes problems by changing the concentration of the medium that the sample is incubated in. When doing upright microscopy, also the resulting condensation of the evaporated liquid can hinder imaging. There are several strategies to minimize evaporation. First, check that a very simple solutions like parafilm or a coverslip with edges greased with Vaseline is not sufficient. Other easy solution is to cover the medium with a layer of mineral or silicon oil, a typical approach in mammalian embryo or organoid cultivation. For questions related to oil and evaporation, we recommend for example this thread on microforum.
Among other, more complex strategies to avoid evaporation belongs a heated lid for your imaging chamber set a few °C above the sample temperature. The disadvantage of heated lid is that the sample is harder to access for manipulation like addition of inhibitors or vital stains. A second approach is using microfluidics, which besides avoiding evaporation ensures also perfusion of your sample with fresh media and an added benefit of using microfluidics is that it minimizes the experimental volume and saves on expensive reagents or potentially precious samples. Getting started with microfluidics for biological imaging does not have to be expensive. One can start with 3D printed molds for PDMS from a standard 3D printer and with a syringe pump.
Thermal drift is an issue coming from temperature gradients in your imaging system, either from the slow temperature equilibration of the whole microscope or the effect of the immersion objective. Autofocus systems help in some cases but best is to minimise temperature fluctuations and avoid the situation when you insert initially a cold sample into a heated microscope chamber. Temperature drift occurs most in systems that are slow to equilibrate, like the large environmental boxes, where the heat is transferred only through heated air. Sample drift in the first 30–60 min of the experiment is common. This problem does not occur when using our VAHEAT device (see below), which can be used without problems in the absence of hardware autofocus.
The next issue is that the standard fluorescence microscopes in your imaging facility are not designed for imaging at extreme temperatures (from the perspective of biology) between 50 and 100°C. But for observation of thermophilic prokaryotes, DNA melting, protein denaturation and other experiments it is necessary to keep your sample at these temperatures sometimes for many hours. The main issue is that standard objectives are not designed to work at these temperatures. They are typically specified for 23–37°C and long-term heating as well as frequent temperature fluctuations, e.g., with an objective heater could damage them because the thermal stress to different components of the objective can cause a misalignment or stress induced birefringence of the optical elements inside. Many researchers choose to use only air objectives, which reduces the resolution. Alternatively, they only heat the sample through a stage insert but then the temperature gradient problem occurs again.
A related physical effect that should not be ignored is the refractive index change of your immersion oil as a function of temperature (around 0.004/10°C), potentially decreasing image quality unnecessarily. Therefore, you should contact your immersion media manufacturer for a special immersion composition that has the correct RI (like 1.515) at your desired imaging temperature, if you plan to image above 37°C. Such high temperature oils are available for example from Cargille.
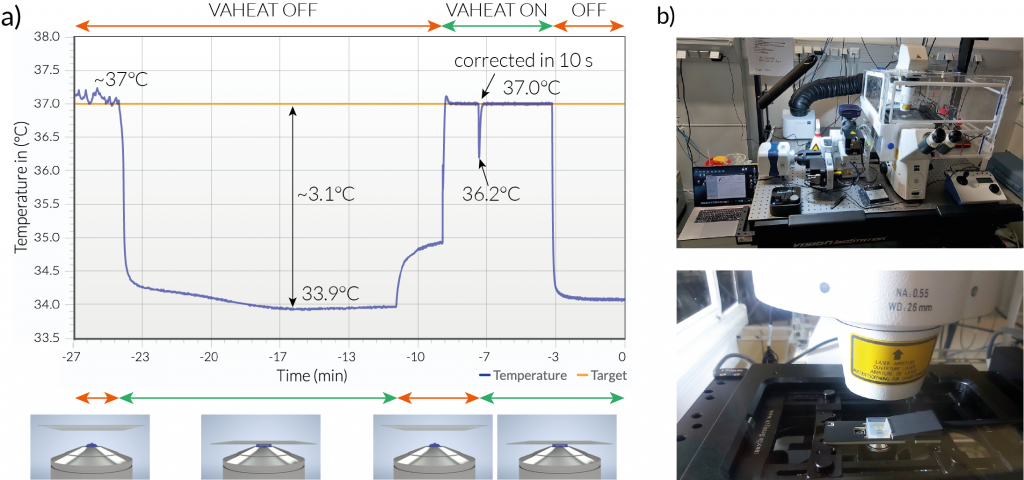
The last issue is very relevant for high resolution imaging. When using immersion oil objectives, your sample is directly thermally coupled to the objective and the microscope body, which typically assumes the room temperature. This causes a permanent heat flow and decrease of your sample temperature by several degrees. In other words, if you set your temperature controller to 37°C, your sample will never be incubated at 37°C and will be sitting in a temperature gradient. We characterized this phenomenon on a standard spinning disk confocal microscope using our VAHEAT temperature control device as thermometer and heater directly in the sample (Fig. 2).
A common approach to prevent the temperature drop in the field of view is to use objective heaters. This approach, however, often leads to a degradation of imaging quality when working with high-NA objectives (NA>1). They are usually designed to work at room temperature, some are specified to operate at 37°C. If you, however, change their temperature beyond the specified range, the optical performance will get worse. In TIRF systems this can even lead to the loss of the critical angle.
The temperature variations inside the FOV are also stressed in this Microscopy U article.
„Among the most significant factors associated with temperature fluctuations is that the microscope stage, frame, and objectives can act as heat sinks and counteract the efforts of the specimen heating system. This problem is compounded when immersion objectives are used because the optical coupling medium, which can be oil, glycerin, or water, has a much higher thermal conductivity than air. Coupled with the close proximity of high numerical aperture objectives to the specimen, as well as the thermal load of the objective itself, the entire system can be rapidly deprived of heat if the objective is not thermally controlled. In the case of the static chambers described above, the area directly under the objective is often up to 5°C cooler than the remainder of the specimen chamber.“
Conventional solutions for temperature control in microscopy
There are several conventional solutions for environmental control. There is no single device that would be a perfect fit for every temperature-sensitive experiment. You have to decide based on your most common application or purchase multiple devices for one microscope system. They can be sometimes beneficially combined together.
1) Large environmental boxes around the microscope. The disadvantages are that the temperature is measured far away from the sample and temperature changes can be really slow. Such microscope in the box takes several hours to equilibrate, therefore it is impossible to run temperature profiles on such system (think a quick heat shock while simultaneously imaging the sample). Slow equilibration also means more prominent temperature-related sample drift compared to other devices. In practice it means that such boxed microscope is often dedicated to imaging at one temperature only, typically at 37°C. Naturally, this environmental box cannot be moved to another microscope. The advantage of this solution is that some environmental boxes can also be cooled to around 10°C and then used in combination with a smaller heating element to reach temperatures below ambient temperature.
2) Stage top incubators/heated stage inserts combined with objective heaters. This is a working but rather complicated and expensive solution when using immersion oil objectives. Because of its complexity, this combination is only suitable for experienced users. Depending on the objective in use, the system needs to be thermally calibrated and if not set right, the two feedback loops of stage top incubator and objective heater can interfere. Such a setup is not suited for temperature profiles (ramps) only for imaging at a constant temperature. It can be necessary to isolate the heated objective from the nosepiece turret, which again acts as the heat sink. Finally, objective heaters might not be suitable for temperatures above 37°C because the objectives are not specified to work at these temperatures, as mentioned earlier. The commercial objective heaters often have an upper limit of 50 to 55°C, which is not enough for some applications. If the stage top incubator is used without the objective heater, then there is a temperature gradient across the sample caused by the familiar heat sink effect of the objective.
When you need efficient cooling as well as heating, you have a choice between Peltier element-based devices and liquid flow-based devices.
3) Peltier element-based devices have an unbeatable temperature range, are quite fast and precise but are hard to miniaturize and there always needs to be a temperature sink connected to the element, like a larger block of metal, which can be impractical.
4) The liquid flow-based devices are quite versatile and fast operating but they are not very user friendly.
5) Another option is to build a temperature controller yourself. It might be worth it for some very special one-off application that the commercial solutions cannot address. DIY in science is generally easier these days in times of open science, YouTube tutorials, Arduino and 3D printing but if your motivation is only to save money by not purchasing a commercial system, we would argue it is not worth the effort and time invested into it and the end result will be probably inferior to the commercially available devices.
Precise temperature control with VAHEAT
The shortcomings of various systems for temperature control described above led us to designing VAHEAT. We believe that temperature control should be as simple as turning on a laser without worrying about calibration or decreased image quality. Our main motivation was to have the actual sample temperature in the field of view under precise control and to design a device, which is suitable for high sensitivity microscopy applications like single molecule and superresolution studies. We chose the heating strategy by transparent thin film element made of indium tin oxide (ITO) and temperature probe built directly into a single chip, the coverslip, on which the sample is mounted, see Fig. 3. Such ITO layer-based heating solutions are best for thermal homogeneity, which is also highlighted in this Microscopy U article.
„For example, chambers featuring coverslips that are heated with a conductive coating often produce superior thermal consistency across the glass surface than do chambers that heat only the metal surrounding the periphery of the coverslip. In the latter case thermal gradients can be substantial. Furthermore, high numerical aperture oil or water immersion objectives can act as a sink to conduct heat away from the specimen chamber and into the metallic objective, thus creating a thermal gradient in the region of the immersion medium.“
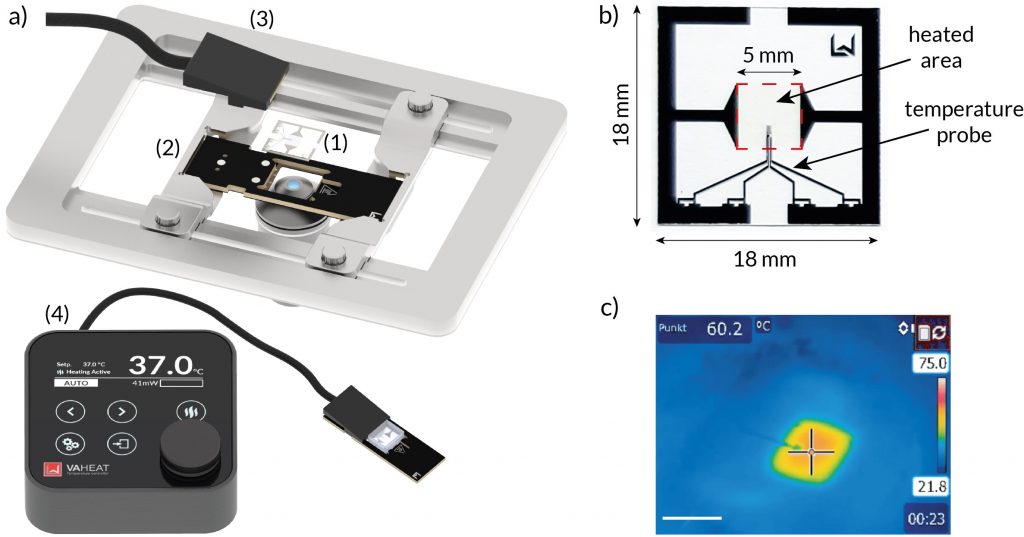
With VAHEAT, the temperature can be changed with rates up to 100°C per second and stabilized well below 0.1°C of set value without any modifications to your microscope. The core of VAHEAT is the Smart Substrates, functionalized microscope glass coverslips, in which the sensitive temperature probe and the transparent heating element are connected into an active feedback loop. The Smart Substrates read and regulate the sample temperature 83 times per second ensuring that it stays precisely at the set value independent of environmental conditions. The Smart Substrates are also available in a version with reservoir for liquid samples. The device allows programming arbitrary temperature profiles similar to a PCR cycler and is compatible with microfluidics. While mainly meant for use with high NA objectives in an inverted configuration, the modularity of the VAHEAT system allows to mount it on the vast majority of optical microscopes.
Since VAHEAT heats only a small volume of the sample, it can be used with high NA immersion oil objectives at high temperatures. There is no issue with overheating of the objective. In one experiment we ran VAHEAT at 75.0°C in contact with a Nikon TIRF 100x/1.49 NA objective for six hours. The objective warmed up by only 5 degrees to 29°C in the first three hours and equilibrated at that point, showing that with VAHEAT one does not have to be afraid of higher experimental temperatures and that VAHEAT is making objective heaters obsolete.
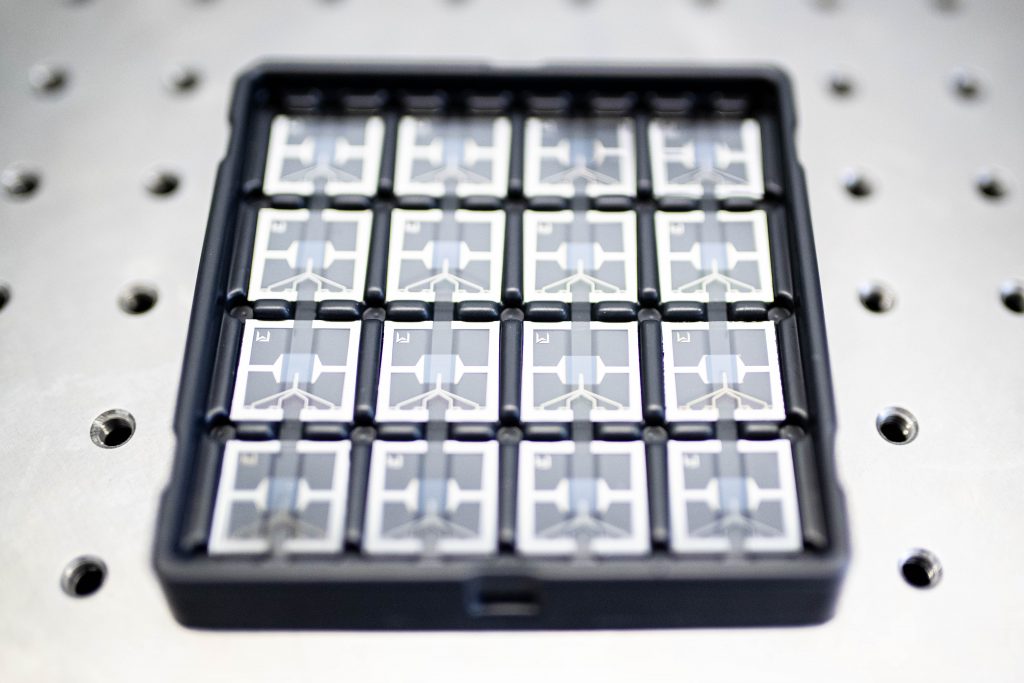
VAHEAT can help all life scientists to perform temperature-sensitive experiments, but for certain fields, like for live imaging of thermophilic microorganisms, characterisation of thermoresponsive polymers or DNA nanotechnology, it is a breakthrough technology, enabling experiments that have not been possible before. The fifty labs and companies, which have started using our device since it was launched in 2020 work in various fields, such as single molecule biophysics, colloidal chemistry and cell biology. They use VAHEAT to study phase transitions, liquid crystals, lipid layers and vesicles and artificial cells, extremophilic microbes, heat shock response or DNA and proteins in solution.
The first publications referencing our device are getting published now. VAHEAT was used for live cell imaging of thermophilic bacteria by the laboratory of Prof. Guillaume Baffou (Institut Fresnel) to study the influence of confinement on bacterial growth1. VAHEAT was used for heat shock and live cell imaging of temperature sensitive alleles in yeast by confocal microscopy in the team of Dr. Wolfgang Zachariae (MPI for Biochemistry) in a research project on mechanisms driving meiosis2. VAHEAT was also utilized in a study using DNA origami to create an artificial macromolecular transport in the laboratory of Prof. Henrik Dietz (TU Munich). The study used single molecule TIRF imaging for detection3.
With the plug and play VAHEAT system it is also very straightforward to export temperature recordings from the experiment. Therefore, we hope that the device not only enables new types of experiments, but also contributes to improved reporting and reproducibility of imaging experiments enabling high sensitivity microscopy for everyone.
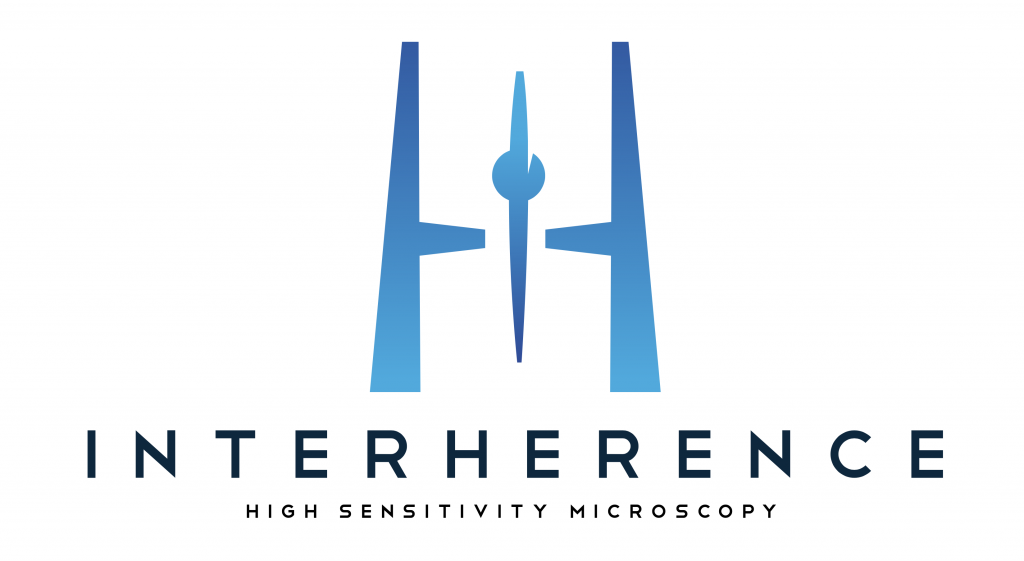
About Interherence
Interherence GmbH is a spin-off from the Max Planck Institute for the Science of Light in Erlangen, Germany. Since 2020 it has been developing the next generation of equipment for high sensitivity optical microscopy in life sciences, material sciences and in the pharmaceutical industry. Its unique products arise from the combination of optics with modern methods of micro and nanofabrication. The miniaturized microscope heating stage VAHEAT is the first product that Interherence brought to the market. It is an enabling technology for microscopy of many temperature sensitive processes that could not be imaged before. The next product will be launched in 2022. Stay tuned for #QUSCITE!
We are happy to discuss any topic related to temperature control in microscopy.
Contact: Dr. Jaroslav Icha, jaroslav@interherence.com, +49 172 208 7522
References:
1. Molinaro, C., et al., Are bacteria claustrophobic? The problem of micrometric spatial confinement for the culturing of micro-organisms. RSC Advances, 11, 12500–12506 (2021).
2. Mengoli, V., et al., Deprotection of centromeric cohesin at meiosis II requires APC/C activity but not kinetochore tension. The EMBO Journal, 40, e106812 (2021).
3. Stömmer, P., A synthetic tubular molecular transport system. Nature Communications, 12, 4393, (2021).