Pointing a tiny telescope at the microcosmos
Posted by Fabian Voigt, on 8 May 2023
I recently published a paper that takes inspiration from both the design of telescopes and the eyes of scallops to come up with a novel way of building multi-immersion microscope objectives (F. Voigt et al, Nature Biotechnology, 2023). Bringing together ideas from fields that usually do not communicate with each other resulted in the most fun project I have worked on so far. What is not described in the paper, though, is the background story of how this project came together – a story that is rooted in my teenage years when I wanted to become an astronomer
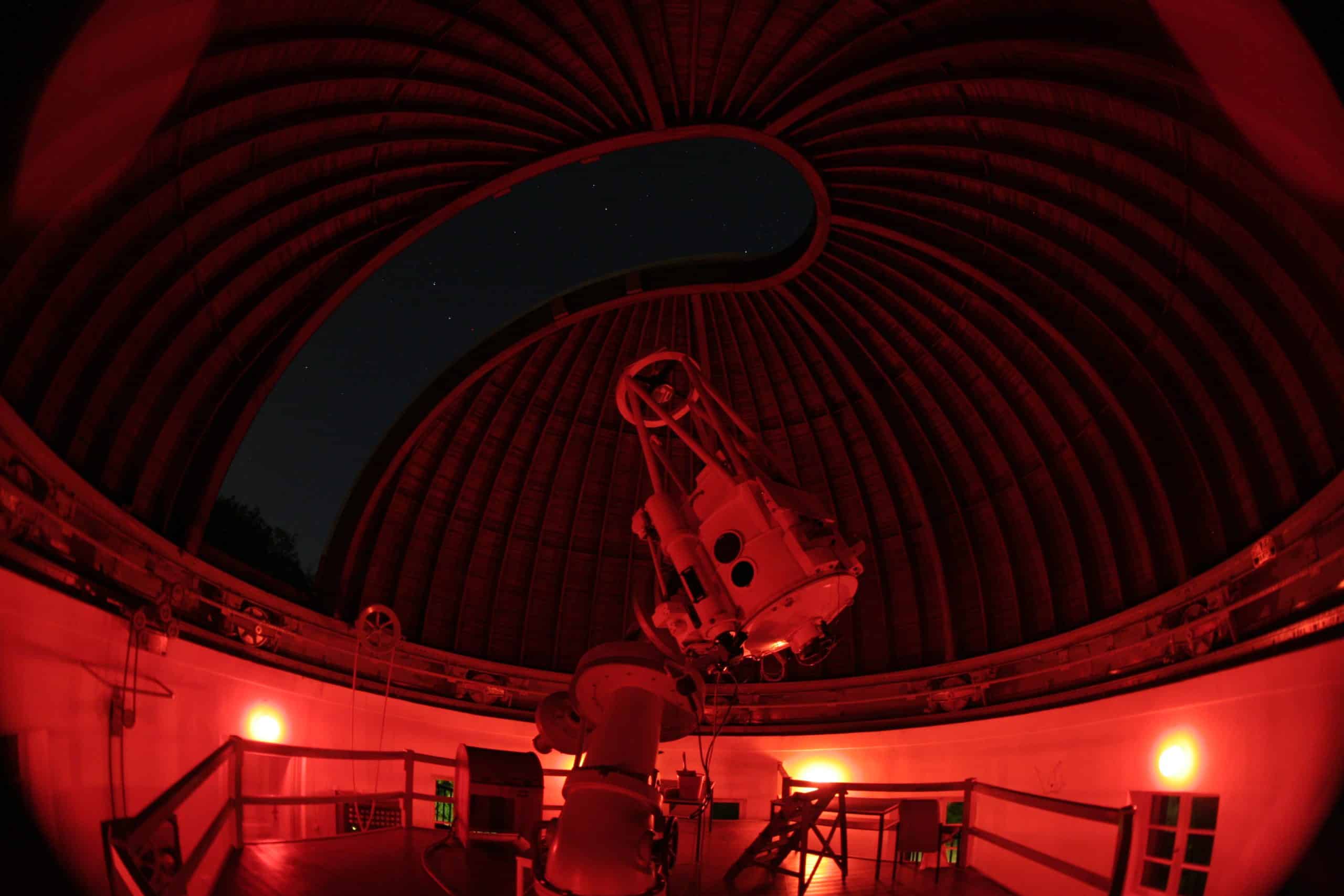
I grew up next to one of Germany’s old observatories – the Babelsberg Observatory (now part of the Leibniz Institute for Astrophysics ). It was just up the road from where I lived in Potsdam, a city close to Berlin. After I had visited the observatory several times, I begged and begged and was finally allowed to use an old Zeiss telescope with 50 cm aperture whenever I pleased in exchange for helping during outreach events. Back in 1913, the Babelsberg observatory was located in the outskirts of Berlin to avoid the stray light of the city – but in the intervening century, the city grew, light pollution got worse and worse; and as a result, the telescopes became unsuitable for professional observations. As a consequence, the 50 cm Zeiss sat unused in its dome. For a 15-year old, this was a golden opportunity: Upgrading from my small amateur telescope to a multi-ton monster with 100x the light-gathering power was a dream come true. Potsdam has around 50 clear nights per year and I tried to getas much observation time as possible, despite the risk of being extra sleepy during the next school day. Using the telescope, I visually observed double stars, globular clusters, the moon, and planets. I vividly remember hunting and finding a faint Pluto back when it was still classified as a planet.
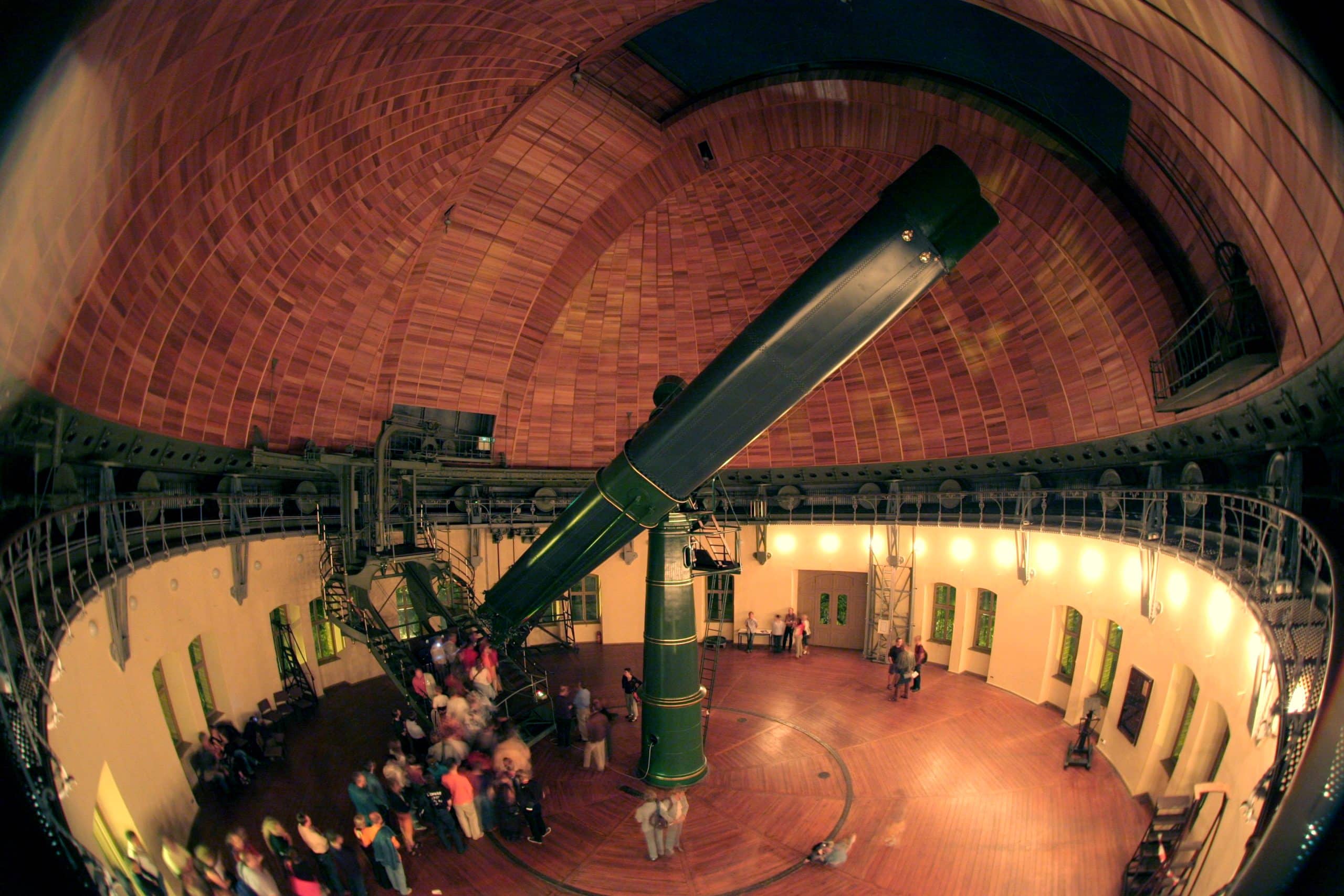
There was a second telescope that I was allowed to use: The Potsdam Great Refractor – a behemoth of a telescope that is actually composed of two lens telescopes in a single tube: A big “photographic” telescope with 80 cm aperture and a smaller visual telescope with 50 cm aperture, both with a focal length of 12.5 meters. The Great Refractor has an enduring legacy in the history of optics: Shortly after its inauguration, it turned out that the optical quality of the objectives were not as good as expected and it took many years to fix the problem. To diagnose the issue, the astronomer tasked with finding a solution – Johannes Hartmann – invented a technique that forms the basis of what is known today as the “Shack-Hartmann” wavefront sensors, which is widely used in adaptive optics. As the company that made the objectives was unable to improve image quality to satisfactory levels, the task of retouching the 50 cm objective fell to the astro-optician Bernhard Schmidt (1879-1935) who successfully turned it into an excellent objective shortly before World War I. This is a story almost unheard of today: A big company cannot fix a problem with their incredibly expensive product and a person working out of a small shed has to save the day. Given that big telescopes were the “Big Science” machines of their age, this is a bit as if a lonely inventor has to be called in in order to fix a broken Large Hadron Collider! However, Schmidt was no ordinary optician: He was a master craftsman who routinely polished optics to a quality level that far exceeded the work of the best optics companies of his time – despite being an amputee who lost his right hand at an age of 15. When trying to build a pipe bomb out of homemade gunpowder, the device exploded prematurely and by the time he reached a hospital, his entire lower right arm had to be amputated for fear of infection. Schmidt left a lasting legacy in the history of astronomy by inventing a telescope design named after him: The Schmidt telescope.
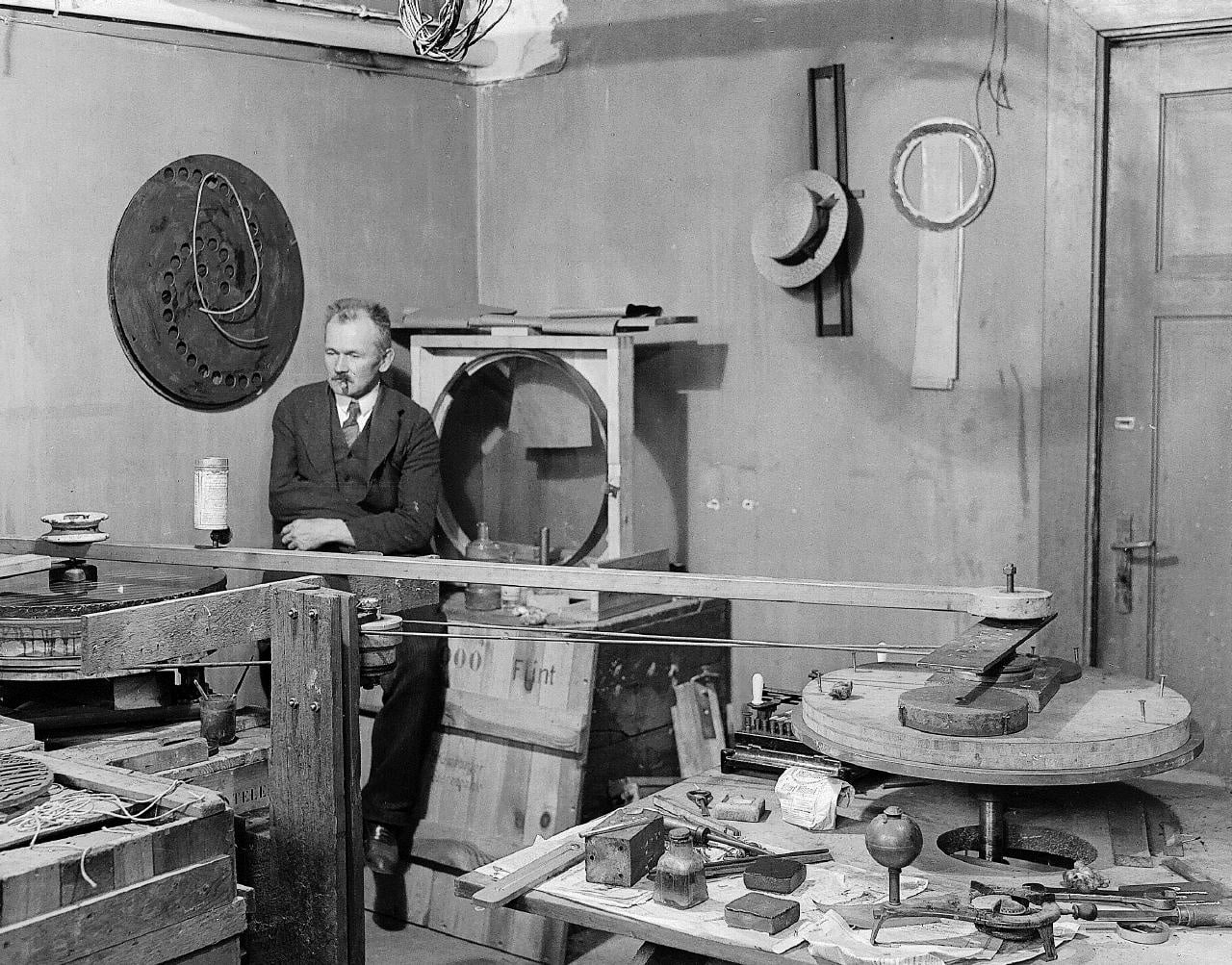
The Schmidt telescope solved a crucial problem that plagued astronomers in the early 20th century: It had become clear that mirror telescopes were superior to lens telescopes because mirrors could be supported from the rear whereas lenses would sag under their own weight. This meant that there was a size limit to the maximum aperture that a lens telescope could have: The bigger the aperture, the more photons can be collected, but around 1 m aperture, the gravity-induced deformations of the optical surfaces would become unbearable. As telescopes get pointed at different parts of the night sky, the image quality is direction-dependent. Mirrors, however, can be supported across their entire rear area, which means that such annoying deformations can be minimized. Reflective telescopes were acknowledged as the way forward by astronomers during the 1920s, but they had a crucial limitation: No one had been able to build a mirror telescope that had excellent image quality over a large field-of-view (FOV). At this time, reflective telescopes only offered good image quality over a small patch of the night sky a fraction of the moon’s size. When trying to scale up the FOV, aberrations such as coma would rapidly degrade the image quality when going away from the optical axis.
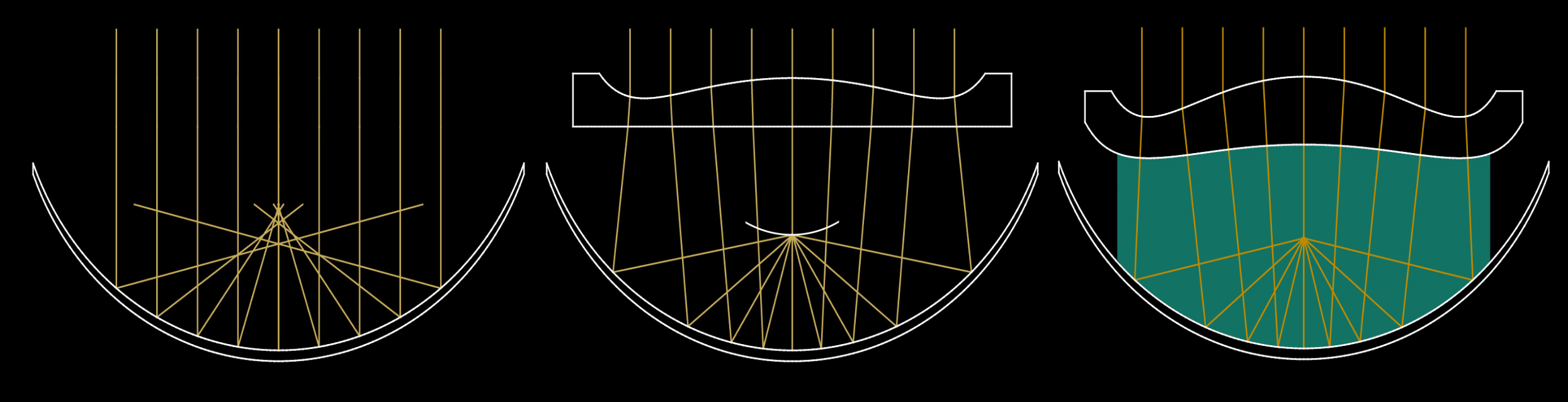
It was Bernhard Schmidt who came up with the solution: By combining a spherical mirror with a weak correction lens, a “correction plate” or “Schmidt plate”, he could achieve excellent image quality over a FOV many moons in size. A spherical mirror is easy to manufacture, however, the correction plate needs to be an asphere: Its shape can not be described by a single radius as in the case of a spherical surface, instead it needs to follow a polynomial curve exactly. Without the correction plate, the image created by the spherical mirror would be hopelessly blurry due to spherical aberration. However when placed in the correct distance from the curved mirror, the correction plate cancels the spherical aberration, resulting in an excellent image quality over a large FOV. Describing an optical system mathematically is one thing, but building and demonstrating an actual prototype is another complexity level. Manufacturing the aspherical correction plate exactly to specifications with 1930s technology is supremely difficult. However, Schmidt came up with a clever solution: He noted that he could arrive at the desired shape with the help of the atmosphere.Schmidt placed a flat glass disk on a vacuum chamber and evacuated the air and due to the pressure differential, the disk deforms. Schmidt realized that for a certain pressure and glass thickness this deformation equals the target polynomial with a residual term describing a spherical surface. Therefore, he polished a sphere into the deformed disk and upon returning to atmospheric pressure, the correction plate had the desired shape. While the idea of the Schmidt telescope became known in the early 1930s, this manufacturing method was a closely guarded secret during Schmidt’s lifetime.
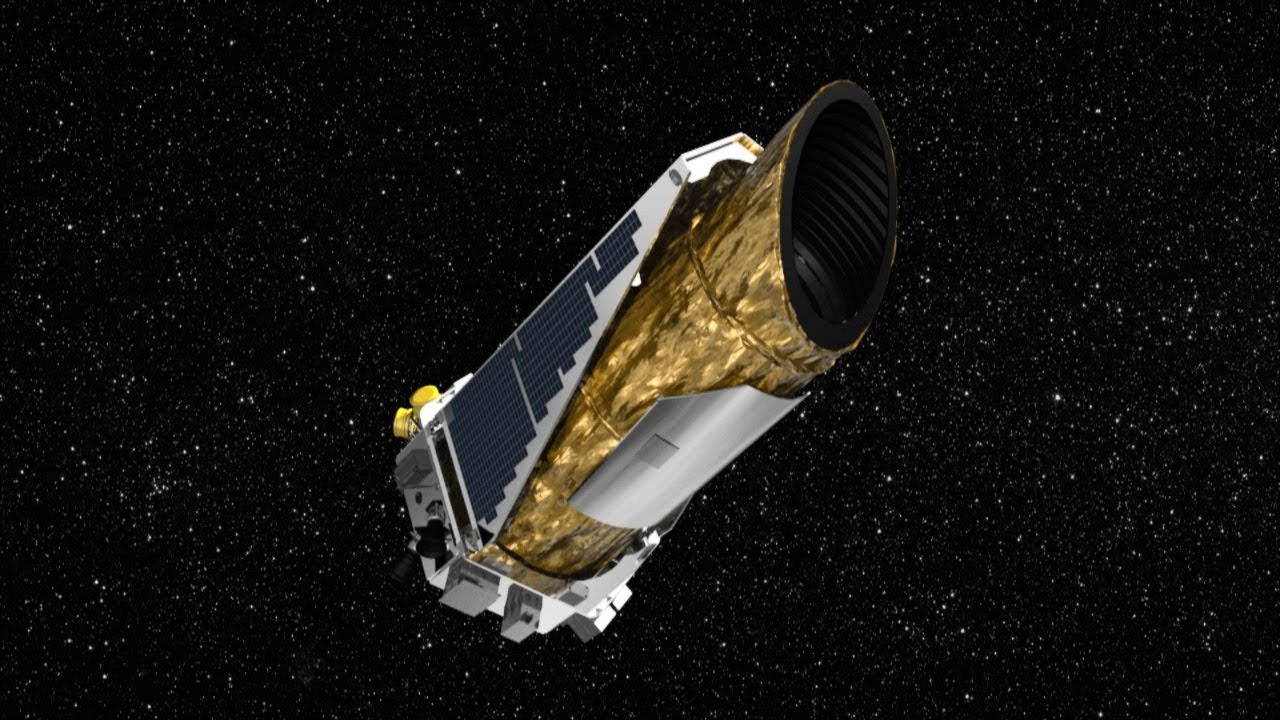
Sadly, he did not live long enough to see the widespread success of his invention. He died in 1935 at the age of 56 and it was only in the late 1940s and 1950s that almost all major observatories around the world started installing telescopes based on his idea. The combination of the high light-gathering power, thanks to a big mirror, and the large FOV makes Schmidt telescopes perfect for surveys of the night sky to this day. For example, the Kepler space telescope operated by NASA between 2009 and 2018 is a Schmidt telescope optimized for detecting exoplanets – planets that orbit distant stars. By the end of the mission, it had discovered more than 2700 planets outside of our solar system, making it the most prolific exoplanet hunter in astronomy.
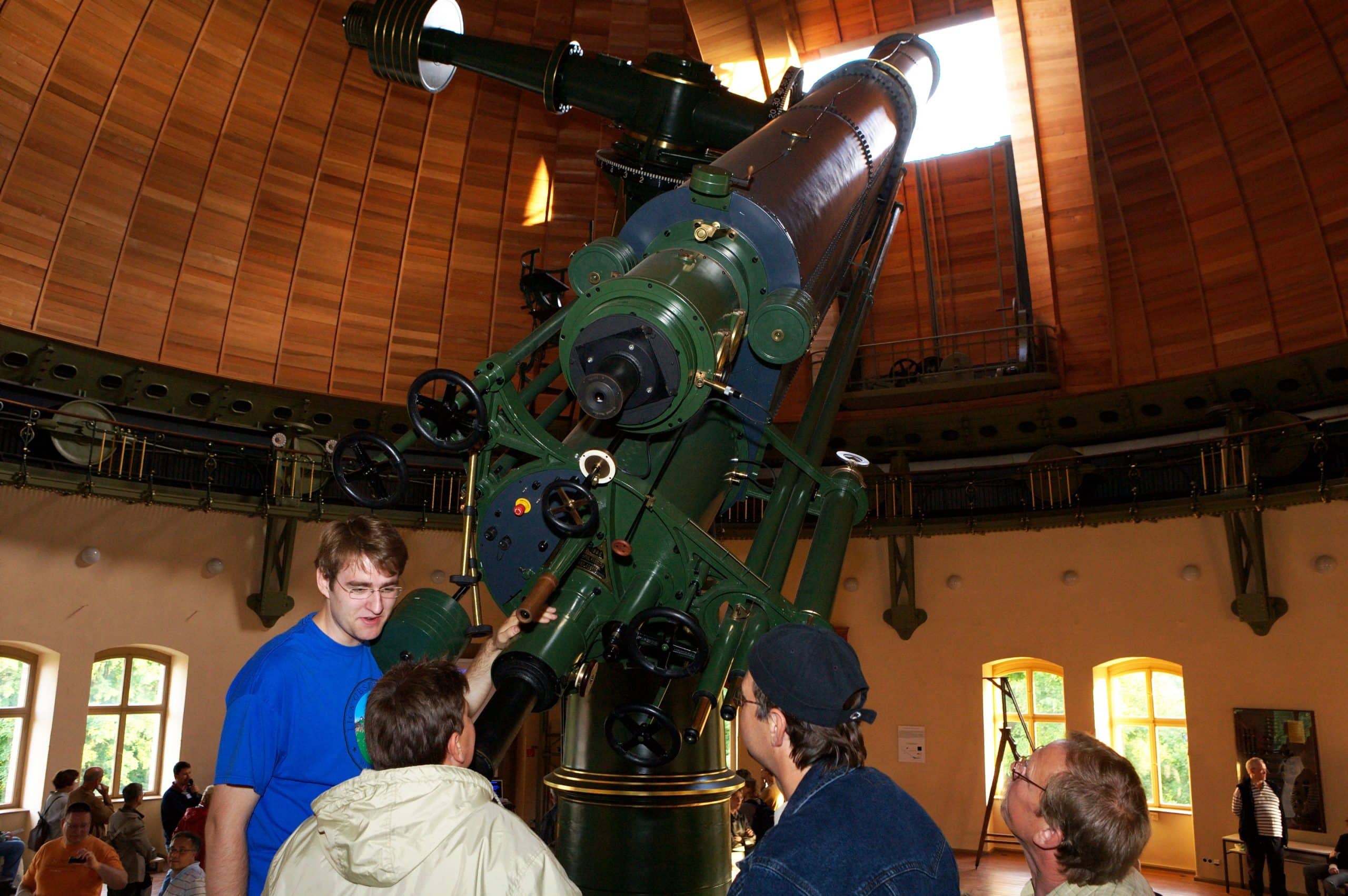
As a young telescope geek, being given the opportunity to observe with the Potsdam Great Refractor and thus an instrument that Schmidt himself polished to perfection, was an amazing opportunity. Even after moving to Switzerland for my undergrad, I regularly helped with outreach events at the Astrophysical Institute Potsdam (AIP). It was also at AIP where an optical designer, Manfred Woche, first showed me the optical design software Zemax that he used to design astronomical spectrographs. Optical design kindled my interest because it is an art and a science: On the one hand, it is deeply mathematical, on the other hand, its history is full of stories like Bernhard Schmidt, who (literally) single-handedly came up with an ingenious way of building an optical system with minimal aberrations. While I considered a career in astronomical optics during my late high school years, I was also fascinated by neuroscience. Unsure what to pursue, I postponed the decision and started studying Interdisciplinary Science at ETH Zurich in late 2007, a BSc program that allowed me to build my own curriculum. Being allowed to choose lectures and seminars out of the entire course catalog of ETH was wonderful, but also daunting. During my first weeks as an undergrad, I went to a physics seminar by Fritjof Helmchen who had just started his lab at the Brain Research Institute at the University of Zurich. Fritjof presented his work on 3D neuronal activity measurements using two-photon imaging and fast spiral scanning and I realized that working on microscopes allowed me to combine my interests in optics and neuroscience. After his lecture I asked Fritjof whether I could visit his lab and I proceeded to do a semester project, my BSc, and MSc thesis and my PhD in Fritjof’s lab – even as an undergraduate student, he offered me enormous freedom in that I was allowed to pick and develop my own projects. During my MSc years, tissue clearing gained more and more interest in the community and better and better protocols were developed and I ended up developing an open source light-sheet microscope for imaging large cleared tissue samples – the mesoscale selective plane illumination microscope (mesospim.org) – for my PhD. Even before the mesoSPIM project began ramping up, I started playing more and more with Zemax to see how one could design custom microscope objectives both for imaging neuronal activity across large FOVs of the mouse neocortex and for imaging large cleared tissue samples. At some point I realized that there is something interesting about reflection in the context of immersion; the way a light ray gets reflected by a mirror does not depend on the refractive index the mirror is in touch with – in other words, the law of reflection does not contain any dependence on refractive index. This means that, in principle, a reflective design can provide excellent image quality when filled with immersion media of different refractive indices without changes to the alignment. Using a mirror in touch with a liquid medium is something that is usually not done in microscope, but I knew that such an approach would work as there is an eye design in nature that uses immersed mirrors: Scallops have 60 to 200 eyes, each of which contains a cup-shaped mirror to concentrate the light on the photoreceptors. Most people do not know that scallops can see – after all, only the strong adductor muscle that closes the valves gets eaten as seafood and the mantle with the eyes never reaches the plate.
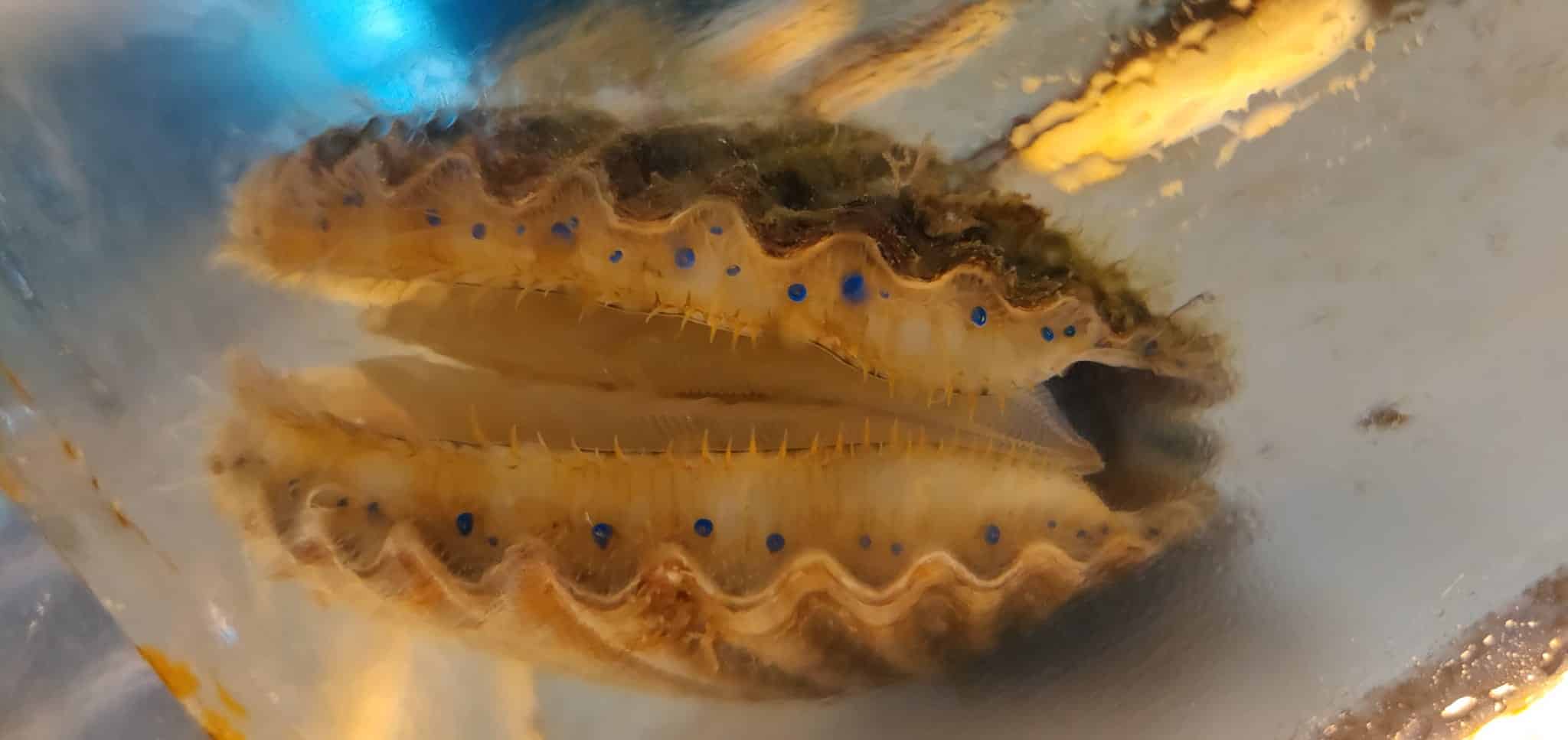
I knew about this after reading the book “Animal eyes” by Dan-Eric Nilsson and Michael F. Land, which is an utterly fascinating read describing all the clever ways evolution came up for sensing light. This was like an existence proof. The concept of an immersed mirror system exists in nature, so there is no fundamental limit in making it work for microscopy. And, who are the masters of using mirrors in optical systems – astronomers with their reflective telescopes! There are all kinds of telescope designs based on mirrors, but what we want in a microscope is first and foremost a large field of view and a large numerical aperture. Most telescopes do not reach high NA’s (or low f-Numbers), but there are a few designs that can go down to f/1 (equivalent to NA 0.5) – and famously, the Schmidt telescope is one of them. It took only a few minutes in Zemax to take a Schmidt telescope, shrink it down to the size of a microscope objective, fill it with a liquid medium, and reoptimize the shape of the correction plate to turn it into an immersion objective. However, there was a problem: While the reflection off a mirror is index-independent, the Schmidt design relies on the refractive correction plate which would form the entrance window to the liquid-filled chamber. Even though the correction plate does not have a lot of optical power, it redirects the passing rays in a way that there is some refraction occurring at the interface between the correction plate and the liquid. The amount of deviation of passing rays depends on the index. As a result, when changing the medium, spherical aberration is reintroduced when the index deviates from the value the correction plate was designed for. Such a “Schmidt objective” is thus an immersion objective, but the correction plate needs to be exchanged whenever a different medium is selected. When playing with such designs in Zemax, I noted that it is possible to change the shape of the inner surface of the correction plate to turn the objective into a true multi-immersion objective. The resulting design has a variety of fascinating features: It is diffraction-limited over mm-size FOVs in air and in any homogeneous immersion liquid. As the numerical aperture scales linearly with the refractive index, the NA of the objective increases when switching to a medium with higher index. This means that the resolution of the objective improves the higher the refractive index of the medium. The product of NA and FOV is constant which means that such an increase is accompanied by a reduction in FOV. After showing this design to Fritjof, he enthusiastically supported the idea and allowed me to embark on this project – even though it meant building high-NA custom microscope objectives in a neuroscience lab with no prior know-how in this niche of optical engineering.
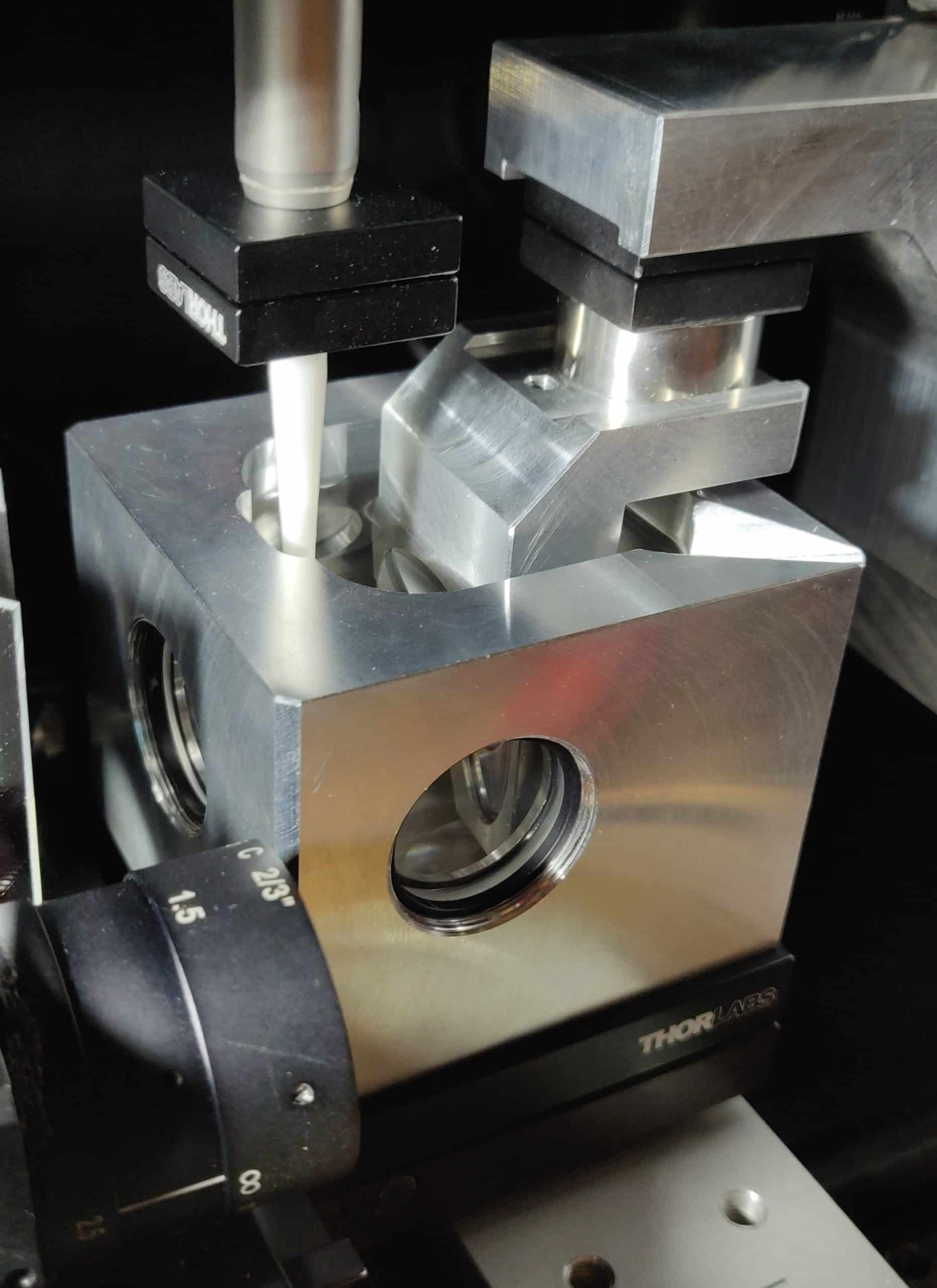
This started a multi-year process of understanding the design, getting the aspheres made, and designing an immersion chamber that would not spill despite being filled with liquids that can creep through the tiniest gaps (BABB, I’m looking at you). The microscope had to be designed, built, and characterized in a way that any deviations from the perfect point spread function could be attributed to what was now the “Schmidt objective”: While Schmidt did not design his system for microscopy applications, the key elements – the spherical mirror and the aspherical correction plate are his invention. The new aspect of my design is the addition of another aspherical surface to make the system multi-immersion capable. After I got the prototype working, I imaged a wide variety of cleared samples ranging from mouse brains, Xenopus tadpoles, chicken embryos, to human brain samples – showcasing that the Schmidt objective is a pretty universal tool to image cleared samples at high resolution. It was also possible to record neuronal activity in the brain of larval zebrafish immersed in water. The Schmidt objective has a longer working distance, larger field of view, higher numerical aperture and wider refractive index range than any existing multi-photon microscope objective. Strikingly, it achieves this with only two optical elements – the mirror and the correction plate – whereas commercially available objectives contain 10-15 individual lenses. This illustrates that building immersion objectives based on reflection unlocks a very interesting design space in optics. While designing lens-based immersion objectives for a wide index range is challenging, by using reflection, the system can be made to work in every homogeneous liquid and in air. I believe that using the Schmidt objective concept, it will be possible to design ‘mesoscope’ objectives: high-numerical-aperture objectives with much larger fields of view than previously thought possible. It is also conceivable to mass-produce less powerful but highly affordable Schmidt objectives for diagnostic applications.
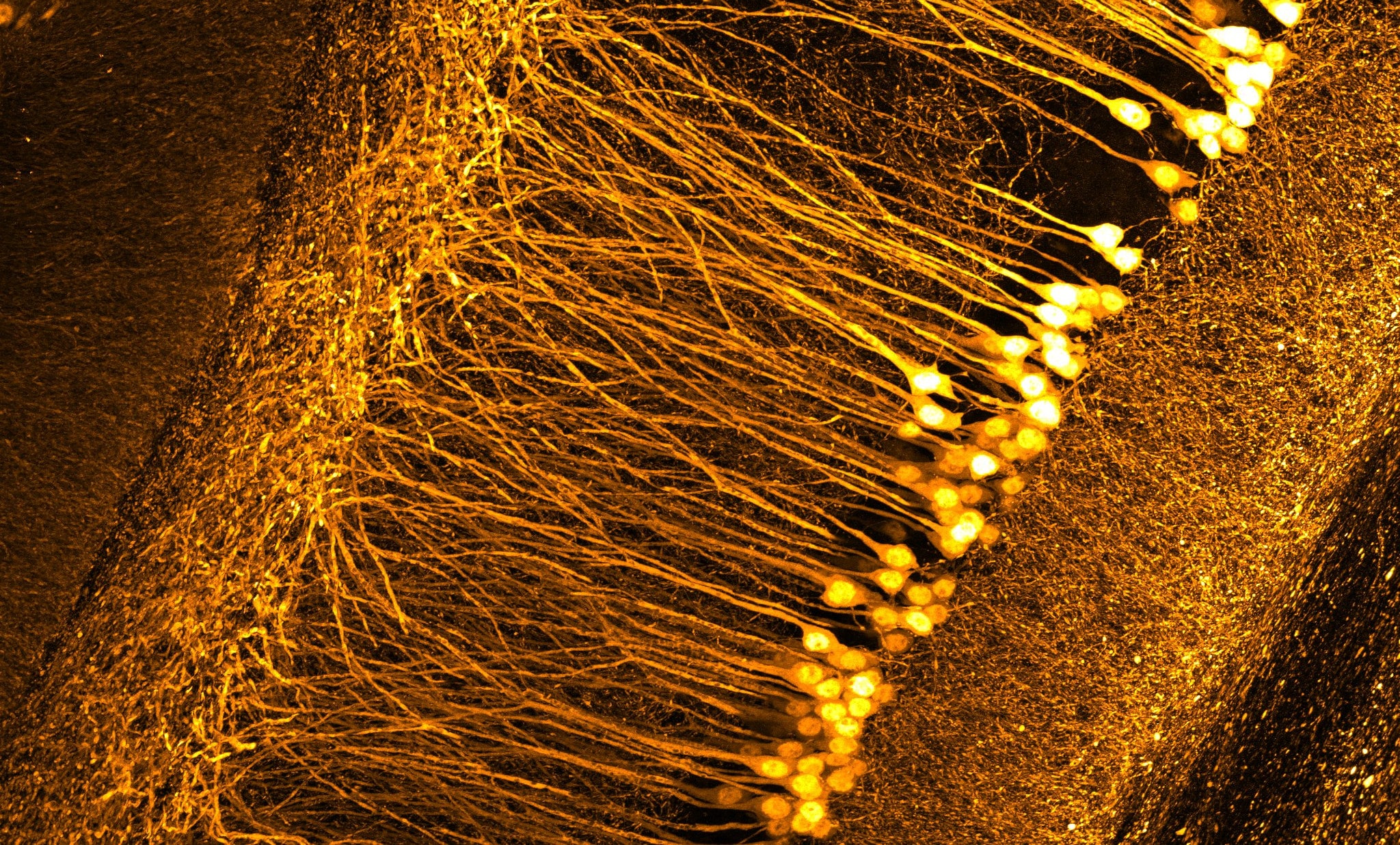
There are some caveats as well: My current prototype is optimized for multi-photon microscopy and not color-corrected for diffraction-limited imaging in the visible spectrum. The reason is that different immersion media differ not just in index, but in their dispersive properties as well. A confocal or light-sheet optimized Schmidt objective needs to be designed with this in mind – but I believe that it will be possible using a custom tube lens. No matter what future Schmidt objectives will look like, they will continue to to be much smaller than their big brother telescopes – unless somebody does whole-body clearing of elephants or a similarly sized sample. In that case, well, maybe we can borrow a mirror from an unused telescope…