Can RNA Be a Master Architect?
Posted by Neelabh Datta, on 29 October 2024
How often do we think of RNA as a mere messenger, shuttling genetic instructions between DNA and proteins, rather than the master architect in the construction of cellular structures? While most are familiar with RNA’s role in transcription and translation, the idea that RNA molecules, particularly long ones, could be responsible for organizing intricate, membrane-less structures within cells might raise an eyebrow. But in the vast arena of cell biology, RNA isn’t just a supporting actor. Instead, it plays a leading role in organizing critical structures like RNP (ribonucleoprotein) granules, which come in various flavors—stress granules, germ granules, and neuronal transport granules, to name a few. These are no ordinary blobs; they are highly dynamic, membrane-less condensates built from RNA-protein interactions.
Now, anyone who’s ever tried to organize a group project knows that too many interactions can lead to chaos (or worse, complete project failure), but in the cellular world, these RNP granules are essential for life. Many of the RNA-binding proteins (RBPs) involved in granule formation have intrinsically disordered regions (IDRs) and prion-like domains (PrLDs)—think of these proteins as having floppy, flexible arms that allow them to hug multiple molecules at once. When these proteins bind to RNA, the combination drives the formation of these multicomponent condensates, much like how a team of builders might assemble a skyscraper.
A recent study from The European Molecular Biology Laboratory (EMBL), by Bose et al. has shown that the assembly of these RNP granules follows a scaffold-client model. Here, RNA-protein interactions are the scaffolds that hold things together, while multivalent protein-protein interactions act as the clients that provide structure. It’s a nice, tidy model that accounts for how these granules form. But here’s the kicker: RNA isn’t just a passive player in this game. It turns out that RNA itself can engage in interactions with other RNA molecules, making it more than just a structural scaffold—it’s part of the construction team, hammer, and nails in hand, helping to build these complex cellular structures.
Take, for instance, the oskar granules in Drosophila melanogaster—yes, fruit flies have a lot to teach us. Oskar granules are phase-separated condensates responsible for packaging maternal RNA and sending it off to the posterior end of developing oocytes (basically baby fruit flies in the making). This RNA oskar, is essential for defining the future body pattern of the embryo. Without these granules, the embryo doesn’t know which end is up (literally). The star player here is Bruno, a protein that binds to specific sequences in the oskar mRNA and drives the assembly of the granules.
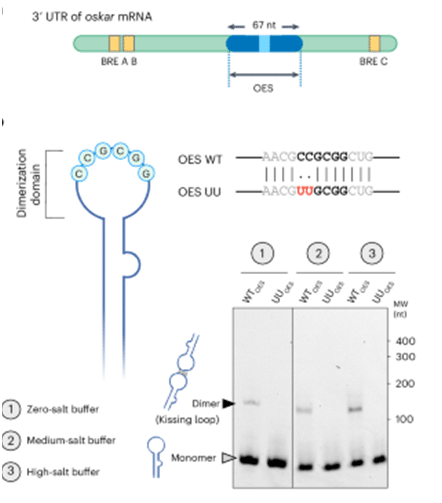
The 3′ untranslated region (UTR) of the oskar mRNA includes key structural elements such as the oskar enhancer sequence (OES) and binding regulatory elements (BREs), with the OES being a 67-nucleotide region containing a palindromic sequence that facilitates molecular interactions. This palindromic sequence within the OES enables kissing-loop interactions between oskar molecules, supporting dimerization that can be observed in both wild-type and UU-mutant OES forms. Image adapted from: https://doi.org/10.1038/s41556-024-01519-3
But before you start imagining Bruno as a lone wolf orchestrating this complex process, know that RNA is not a silent partner. Our friendly oskar RNA forms kissing-loop interactions—yes, it’s as adorable as it sounds—with other RNA molecules. These RNA-RNA interactions, specific to small palindromic sequences, are critical to stabilizing the granules. Without them, it’s like trying to build a Jenga tower with wet noodles. This type of interaction is well-studied in viruses like HIV, where the genome forms dimers via a kissing-loop, crucial for the viral life cycle. In the oskar granules, these kissing loops prevent the granules from falling apart, providing stability that Bruno alone can’t offer.
Here’s where things get particularly interesting (and where you might start to appreciate the mind-bending complexity of RNA). When scientists swapped out the oskar sequence with a scrambled version from HIV-2, the granules didn’t form as well. This suggests that it’s not just the base composition of the RNA that matters, but the actual sequence and its geometry. Imagine trying to fit square pegs into round holes—the geometry of these RNA sequences matters at the molecular level, allowing them to interact just right.
RNA, therefore, isn’t just holding hands with proteins—it’s actively making out with other RNAs (metaphorically, of course) to form these highly organized structures. What’s more, these specific interactions between RNA molecules play a major role in determining which RNA species get included in the granules. It’s like an exclusive party: only the right RNA molecules get past the bouncer, while others are left on the outside. And for oskar, this selectivity is critical because mixing it up with other maternal RNAs would be disastrous for embryonic development.
The role of RNA in granule formation is more than just fascinating biology. It offers a window into how cells manage to compartmentalize different processes without relying on membranes. These condensates can behave like liquids or gels, depending on the RNA-protein ratio, and their physical properties are essential for regulating functions like RNA translation. Disrupting these interactions, particularly the kissing-loop ones, messes with oskar’s ability to repress translation at the right time and place—a bit like showing up with balloons to a funeral. Timing and location are everything when it comes to gene expression.
But wait, it gets even better. The lessons learned from oskar granules are relevant beyond fruit flies. Other organisms, like the fungus Ashbyagossypii, form similar granules with mRNA species that localize to specific parts of the cell. In Ashbya, altering the structure of one mRNA (CLN3) causes it to co-mix with other granules, proving once again that RNA structure is vital in maintaining the specificity of these condensates. Even in the human body, non-specific RNA-RNA interactions abound, especially when mRNAs are released from polysomes under stress. However, unlike the strong, specific RNA kissing-loops, these non-specific interactions are like the flimsy glue that can’t hold the granules together under pressure.
What does all this mean for our understanding of cellular condensates? It’s becoming increasingly clear that RNA is not just a passive player in the assembly of these structures—it’s an active participant, sometimes even taking the lead role in organizing complex RNA-protein networks. The interactions between RNA molecules, particularly those involving specific structures like kissing-loops, add a layer of architectural complexity that we’re only beginning to appreciate.
So, what other roles might RNA be hiding up its sleeve? Is it possible that RNA’s ability to self-assemble and scaffold condensates could be harnessed for therapeutic purposes, such as targeting specific RNA molecules in diseases like cancer or neurodegeneration? One thing’s for sure: the next time someone calls RNA a “messenger,” remind them that even messengers can build empires—one kiss at a time.
Original Paper
Bose, M., Rankovic, B., Mahamid, J. et al. An architectural role of specific RNA–RNA interactions in oskar granules. Nat Cell Biol (2024). https://doi.org/10.1038/s41556-024-01519-3