Highlighting signalling biosensors 1.
Posted by Greg Redpath, on 16 January 2025
Why there is interest in signalling biosensors, considerations for using biosensors, and the original biosensor, GFP.
Why write about signalling biosensors?
In my first postdoctoral position, I was introduced to the concept of endosomal signalling- a way for receptor signalling outcomes to be changed by delivering a signal to different regions of the cell. I found the idea fascinating that a receptor wasn’t just signalling at the plasma membrane, then internalised by endocytosis to terminate the signalling. Endocytosis does so much more than stop signalling, it diversifies it. I also found out very early on that trying to understand what was going on with endosomal signalling was technically very difficult. When you move away from the cell surface, the speed of receptor trafficking, how long a receptor stays in a specific endosomal compartment, and where the receptor ends up, all add layers of complexity to the whole picture. Wanting to understand these layers of complexity in endosomal signalling and its regulation is where my interest in signalling biosensors started.
A signalling biosensor is typically a fluorescent protein based fusion protein that is sensitive to signalling events in the cell (for example, calcium release, kinase activation, enzyme recruitment). The overall aim of using a signalling biosensor is to understand when, where, and what signalling is occurring in the cell (or even the whole organism, but that is beyond the scope of this article and my skills!). Signalling biosensors are often recruitment or intensity based (‘intensiometric’). They respond in a dynamic fashion to signalling events, which means that they can be used in live cell experiments as a read out for signalling in real time (although there have been some cool single molecule biosensors developed for fixed cell applications as well).
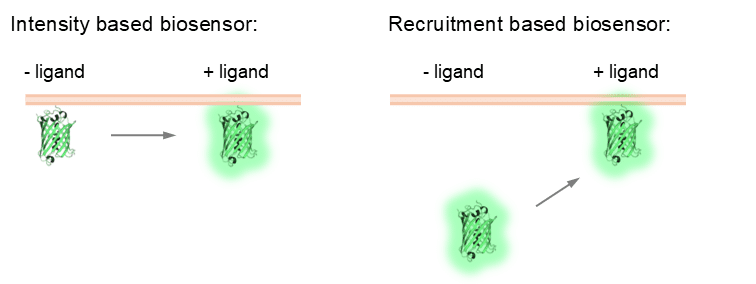
My focus in these articles will be on biosensors used to quantify the outputs of receptor signalling, highlighting considerations when using biosensors, different classes of biosensors and their pros and cons, how biosensors have been used to spatially resolve signalling events (endosomal signalling, yay!) and some recent developments in the biosensor world. I won’t be trying to cover every single biosensor in existence (Eric Greenwald and the Jin Zhang lab have done an excellent job of this with the fluorescent biosensor database: https://biosensordb.ucsd.edu/index.php). Also, for those who want a deep dive on all things biosensors, two highly detailed reviews have been recently published that cover all aspects of signalling biosensors, by Minji Wang, Yifan Da and Yang Tian: 10.1039/d2cs00419d; and Michelle Frei, Sohum Mehta and Jin Zhang: 10.1146/annurev–biophys–030722–021359.
Considerations for selecting and using signalling biosensors.
In cellular imaging, what are we ultimately trying to achieve by using signalling biosensors? We want to be able precisely determine when and where signalling is happening in the cell, and to characterise what this signalling is. For the most part (but not always), this requires live cell imaging to couple where the signalling occurs, when it occurs, and what the signal is. Below I will highlight some important considerations when looking to use signalling biosensors, focussing on the cellular imaging considerations, however, it is always important to ensure the biosensor is specific to the process you are studying first!
Channel availability. As with all fluorescence imaging, the wavelengths or ‘channels’ that are available for imaging is a fundamental consideration. Fluorescence resonance energy transfer (FRET)-based biosensors require the use of two channels (typically cyan/yellow, CFP/YFP, or green/red), which can limit imaging options. For example, if you want to image your receptor using a red fluorescent protein, this in practice only really leaves one channel for biosensor imaging, green. While blue fluorescent protein-based biosensors exist, these typically require UV light to excite them, which cells won’t tolerate for too long and will lead to rapid bleaching. A cyan/yellow FRET biosensor could be used, but this would require care as the emission from YFP could excite the red fluorescent protein. Having single channel biosensors creates options for visualising your receptor, subcellular compartments, or having multiple biosensors in a single experiment.
Bleaching. As with all cellular imaging, bleaching is the bane of imaging biosensors. Bleaching is the loss of fluorescence of a fluorescent protein (or molecule) as it is exposed to the exciting laser/light source over time. A biosensor must be excited with enough light to produce enough signal to quantify its response to receptor activation/ligand stimulation, but with the lowest amount of light possible to achieve this to minimise bleaching. This is especially important when trying to investigate signalling at intracellular/endosomal compartment. If we are just seeking to image the initial, plasma membrane signalling response, the power of the excitation light can be ramped up as we will typically only image for a couple of minutes to capture the signalling. If we want to also catch endosomal signalling, we will be imaging much longer, likely at least 30 minutes. Therefore, to best be able capture these signalling events, biosensors that are relatively photostable and resistant to bleaching are important.
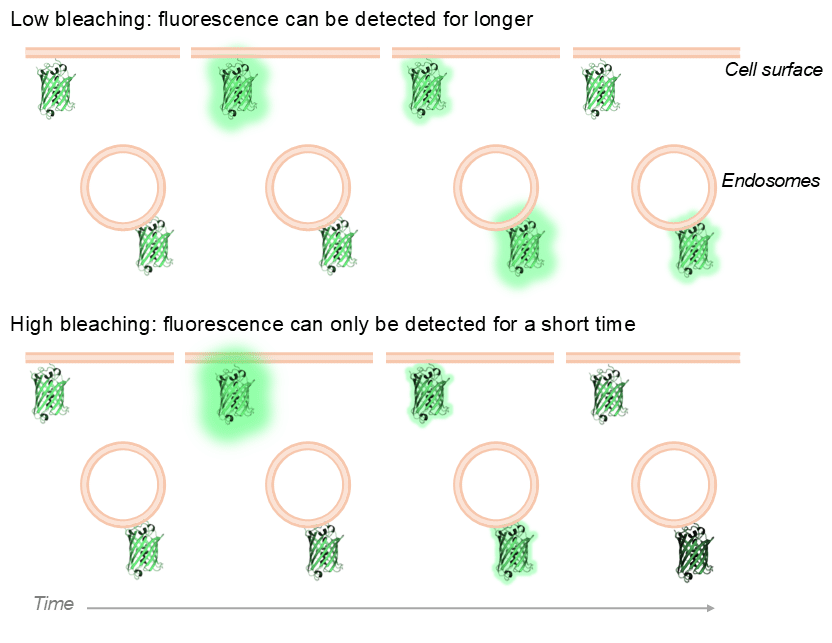
A schematic example of biosensor bleaching. A low bleaching scenario (e.g. low illumination power or bleach-resistant fluorescent protein) enables signal to be detected for longer, and allows the capture of signalling events from other subcellular compartments (e.g. endosomes). In a high bleaching scenario (e.g. high illumination power or bleaching-prone fluorescent protein), plasma membrane biosensor signal can still be detected, but detection of signals occurring later in time (e.g. endosomal signalling) will be hard to detect.
Dynamic range. The dynamic range for the biosensor, that is the range of fluorescence change from baseline to maximal excitation, is a crucial consideration for practical biosensor use. Calcium biosensors typically have fantastic dynamic range. For example, the red calcium biosensor R-GECO has a relatively high Kd for calcium (1200 nM) and a 33x intensity change between basal and maximal fluorescence. Practically, this makes the biosensor useful as even small changes in calcium will result in a quantifiable change in biosensor fluorescence. On the other hand, if a biosensor has low dynamic range, large amounts of ligand binding/receptor activation may be required to elicit a quantifiable signal from the biosensor, but this will not capture more subtle signalling events or signalling events may not be quantifiable with subcellular resolution.
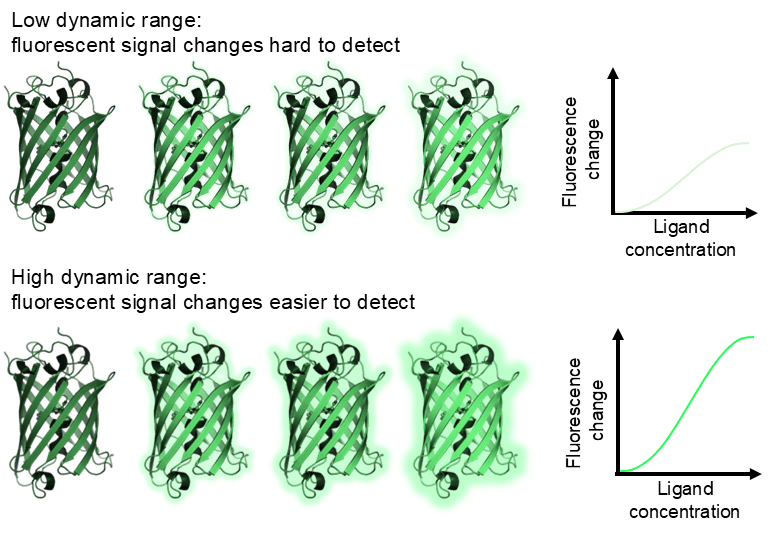
Recruitment ratio. For a recruitment-based biosensor, dynamic range isn’t a factor as the total fluorescence of the biosensor doesn’t change, what does change is its localisation. However, something you do have to consider is the recruitment ratio which could be viewed as a variant of the dynamic range consideration. That is, the ratio the biosensor that is recruited to a compartment when activated compared to its basal localisation. Many recruitment biosensors are cytoplasmic at baseline, then upon ligand stimulation recruit to the plasma membrane or endosomal compartments, depending on where the receptor is active. The ratio of recruitment to the compartment of interest compared to basal localisation is crucial. A high recruitment ratio where the change in localisation of the biosensor from, for example, the cytoplasm to the plasma membrane, is pronounced this makes subsequent quantification easy, but if this ratio is low due to minimal recruitment of the biosensor to the compartment of interest, these recruitment events will be difficult to visualise and quantify.
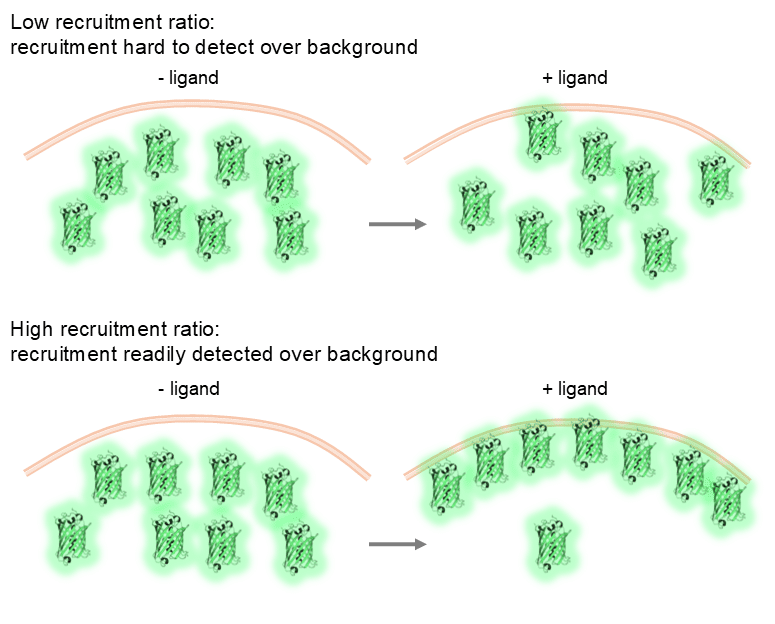
A schematic example of the ratio of biosensor recruitment. In a low recruitment scenario (left), the GFP recruited to the membrane is difficult to distinguish from the cytoplasmic signal. In the high recruitment scenario (right), the GFP recruitment to the membrane is easy to distinguish from the cytoplasmic signal.
The earliest biosensor: GFP.
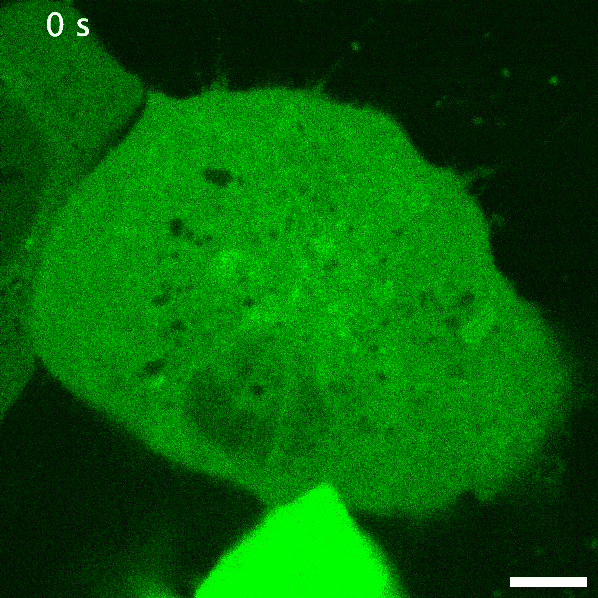
Green fluorescent protein is probably the earliest biosensor developed for imaging purposes. By attaching GFP to a protein of interest, we can readily image where it goes. If GFP- or any fluorescent protein- is attached to a protein that responds to or enacts a signalling event in the cell, the fluorescent protein acts as an indirect readout of the signalling: this is a recruitment biosensor. An example of this is with protein kinase C (PKC). PKC is typically in the cytosol, but upon receptor stimulation by a ligand can translocate the plasma membrane to phosphorylate the receptor and other proteins. When fused to GFP, the plasma membrane translocation of PKC can be readily observed (see the GIF on the right). The timeframe of this membrane translocation gives a good indication of where PKC is active in response to ligand stimulation of a receptor.
Another nice example of fluorescent protein fused biosensors are the mini-G proteins. Mini-G proteins are minimal variants of G protein alpha subunits that are cytoplasmic and form stable complexes with G protein coupled receptors upon activation. The cytoplasmic nature of a mini-G protein means translocation to receptors/membranes can be observed upon ligand stimulation of a receptor, making them recruitment biosensors. Mini-G proteins also highlight the ability of biosensors to detect endosomal/intracellular signalling. Some great imaging form the Stoeber lab highlights how mini-G proteins can detect likely G protein activation at the plasma membrane and intracellularly at the Golgi apparatus, and reveal the location of G protein recruitment is different dependent on the opioid used (10.1126/sciadv.adf6059). This work highlights the power of signalling biosensors- we can start to see not only when signalling occurs, but where it occurs as well!
Pros: The pros of GFP (and fluorescent protein) recruitment biosensors are based in their simplicity. Firstly, they use only a single channel. This allows multi-channel imaging with your receptor of choice, subcellular compartment of interest to verify the location of recruitment, or with another biosensor to characterise multiple signalling events in a single cell. Secondly, the fluorescent protein can be readily changed. This allows the sidestepping of some difficulties associated with biosensor imaging. In situations where bleaching is problematic, a more photostable fluorescent protein can be used. If the cells are particularly sensitive to phototoxicity, a brighter fluorescent protein can be used and laser power minimised when imaging. Finally, imaging and analysis can be kept simple, making recruitment biosensors a great place to start.
Cons and considerations: The drawbacks of GFP/FP based biosensors are the indirect nature of their detection of signalling events, and the potential difficulty with quantifying recruitment if they have a poor enrichment ratio. Most recruitment biosensors are indirect in their very nature. Let’s look at the above example, PKC. PKC phosphorylates targets to affect signalling. If we see PKC recruiting to the cell surface following receptor activation by a ligand, we can surmise it is likely active. However, this is just PKC fused to GFP, nothing about this tells us if PKC is in an active conformation or is a readout of receptor phosphorylation. It is therefore an indirect measure of PKC activation, unlike other PKC biosensors I will detail in my later article. The other potential drawback of recruitment biosensors is quantification when the ratio of recruitment to the compartment of interest is low. In such cases, simple quantification of intensity change on the compartment of interest may not suffice, alternative biosensors may need to be used, and results validated with further methods.
In my next posts, I will talk about FRET, dimerisation dependent fluorescent protein, and circular permuted fluorescent protein biosensors, single molecule biosensors, interesting uses of these biosensors and where the near future may lie in biosensor development.