Primed conversion: the Basics
Posted by Konstantinos Kalyviotis, on 15 November 2021
It has been a bit more than 6 years since the first “primed conversion” article1 was published in May 2015 and around 3 years since I first heard about this new photoconversion technique. I recall that it was almost 1 year after I got my MSc in Greece when I contacted Dr Periklis (Laki) Pantazis to express my interest in pursuing a PhD in his lab. We discussed a lot and he explained to me the basic research pillars of his lab:
- primed conversion – the new kid on the block for photoconversion2
- GenEPi – the force sensor3 and
- bioharmonophores – the biodegradable Second Harmonic Generating nanoprobes4.
The idea for me was to focus on primed conversion. So, we started from the basics.
Photomodifiable fluorescent proteins
Photomodifiable fluorescent proteins (FPs for short) are a subclass of FPs with photochromic characteristics. Proteins of that class can irreversibly or reversibly change their spectral properties (excitation and emission profiles) upon (typically) high-energy light illumination. We can say that there are 3 main categories of photomodifiable FPs:
- photoactivatable FPs – change from a non- or weak- fluorescent state to a nice bright one (see Patterson’s photoactivatable GFP5),
- photoswitchable FPs – can reversibly change between 2 fluorescent states upon light illumination (see Dronpa6) and
- photoconvertible FPs – their excitation and emission spectra change in an irreversible way upon typically 405nm light illumination (see Dendra27).
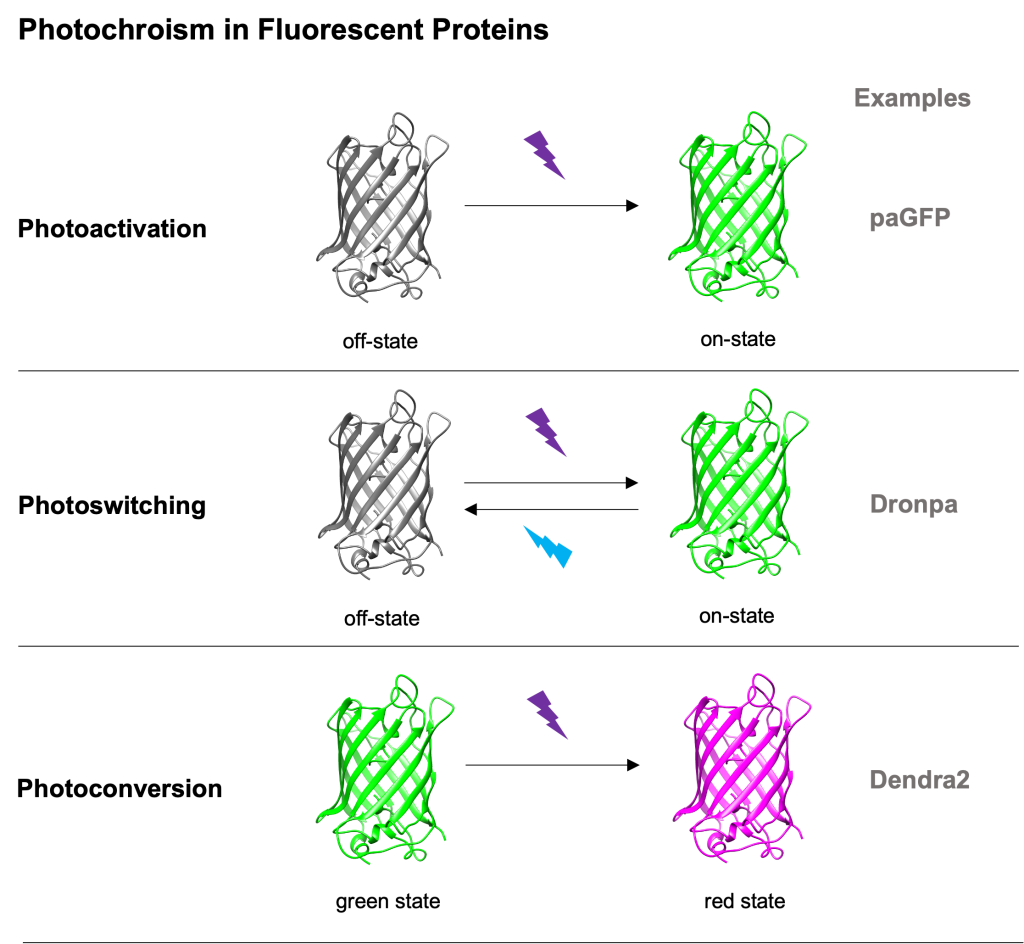
Photoconvertible fluorescent proteins and traditional photoconversion
Although all the photomodifiable FPs can be useful in different experimental setups, photoconvertible FPs have a unique feature which can be of great importance when labelling cells or proteins of interest for tracking purposes. Photoconversion allows for the fluorophore imaging of both the non-converted (green) and converted states (red) while ensuring that no misunderstanding will occur due to the irreversible nature of photoconversion. Thus, both global (green FPs) and sparse (red FPs) labelling can be achieved, increasing at the same time the fidelity of tracking, and lowering the chance of error8,9.
However, traditional 405nm photoconversion is limited by its high energy nature. As traditional photoconversion is a 1 high-energy-photon process, it is rather difficult for someone to achieve single cell photoconversion (mainly in the z-axis) especially in a complex 3D environment with a lot of packed cells, such as the developing zebrafish embryo. Unfortunately for us all, the energy above and below the focal plane where the 405nm light is focused, is usually more than sufficient to photoconvert cells that we would prefer to remain non-converted.
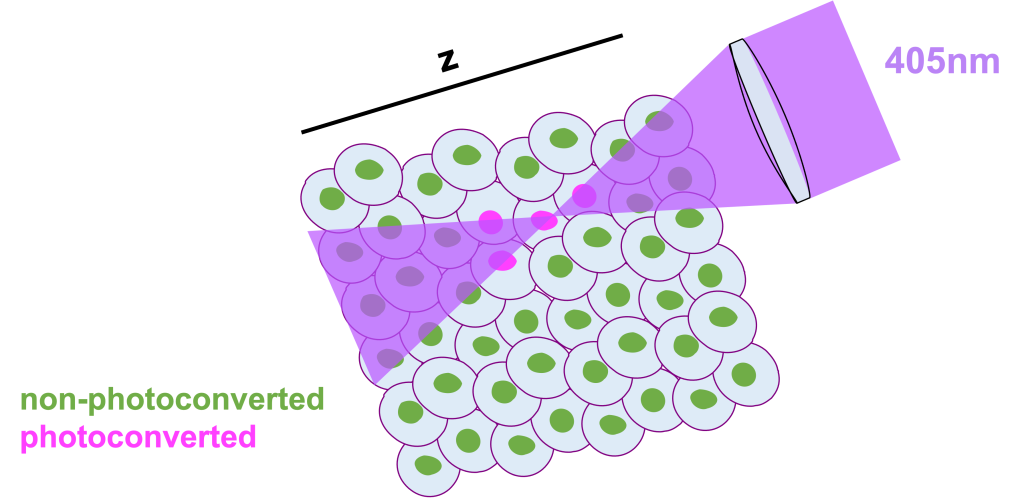
Primed conversion
As mentioned before, it is more than 6 years since primed conversion was first published as a novel and fundamentally different method for axially confined (yay!) and less phototoxic photoconversion (double yay!). The main difference of primed conversion compared to traditional 405nm photoconversion is that primed conversion is a dual illumination process. In primed conversion, the simultaneous illumination of a photoconvertible FP, such as Dendra2, with blue (488nm) and near-Infra-Red (nIR, 730nm) light leads to spectral changes similar to traditional photoconversion. Simpler said, the same red species are produced by using either primed conversion or traditional photoconversion, yet with the added benefit of axial confinement for primed conversion (kill two birds with one stone!).
The idea of how primed conversion works was rather simple at that time. The blue (488nm) beam excites the chromophore which enters a relatively long-lived (~5ms) “primed” state. Then, this intermediate “primed” state absorbs the nIR (730nm) light and the chromophore change happens. Around 2 years of work was needed to better understand the nature of this long-lived state. Manuel, a PhD student of the lab who was working on primed conversion at that time, conducted a series of experiments to better understand the nature of primed conversion’s “primed” state. To this end, he either removed or increased oxygen (Ο2) levels in the protein buffer during primed conversion. Notably, when oxygen was removed from the buffer by saturating it with nitrogen (Ν2) the lifetime of the intermediate state increased, whereas the opposite was observed when oxygen concentration was increased. Considering these observations and the fact that other previously proposed mechanisms fell short to explain such a kind of long-lived intermediate state, the previously uncharacterised “primed” state was now identified to be a T1 triplet “dark” state (dark as no photons are emitted)10. Interestingly, this T1 triplet state can absorb a wide range of red to nIR light, spanning from ~600-850nm; please note that even a good HeNe 633nm laser could work in a simple confocal laser scanning microscope in combination with the right protein.
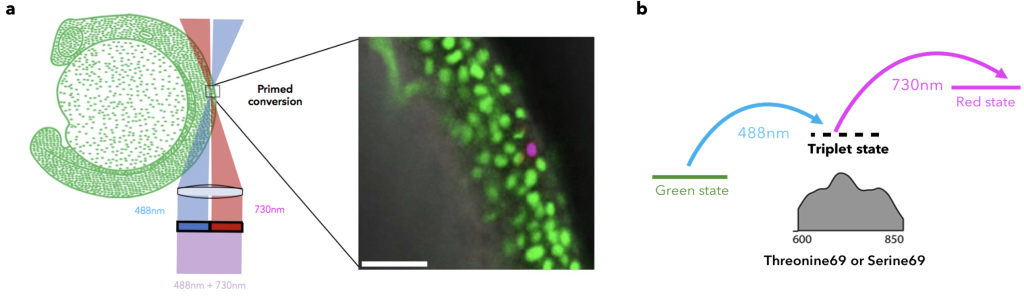
Primed convertible fluorescent proteins (pr-FPs)
Primed conversion was first observed as a feature of the green-to-red photoconvertible FP Dendra2. The characterisation of the “primed” state as a triplet state initiated a series of directed mutagenesis experiments which led to an amazing discovery; primed conversion can be engineered in a fairly simple way10,11. The substitution of the 5th amino acid after the chromophore triplet (HYG) to Threonine or Serine can facilitate primed conversion and renders a green-to-red photoconvertible FP primed convertible or the opposite. For example, the substitution of Threonine74 in Dendra2 to another amino acid, such as Valine, renders Dendra2 non-primed convertible, whereas the substitution of Alanine69 in mEosFP to Threonine, renders mEosFP primed convertible (pr-mEosFP). Using this knowledge, we now have an extended platform of primed convertible FPs (pr-FPs) with different characteristics, such as brightness and oligomerisation states. Our current primed conversion FP toolkit even contains calcium indicators, such as GR-GECO1.2, and optogenetics tools, such as PhOCl10, 12. Overall, the extended pallet of pr-FPs gives us -the biologists- a series of advanced imaging tools that can be used to understand better cellular dynamics.
Labelling of single neurons in zebrafish
One of the most impressive applications of primed conversion since its discovery is probably the labelling of single neurons in the developing zebrafish embryo13. By expressing Dendra2 in the cytoplasm of neurons using a neural-specific promoter and primed converting single neurons, our lab revealed cells’ morphology in zebrafish trigeminal sensory ganglion, optic tectum, and spinal cord. The monitoring of the cytoplasmic diffusion of the photoconverted red form of Dendra2 throughout the entire cell into the neurites allowed for dissecting information about the single neuron branched morphology, highlighting primed conversion as an attractive tool for monitoring neurites dynamics in vivo.
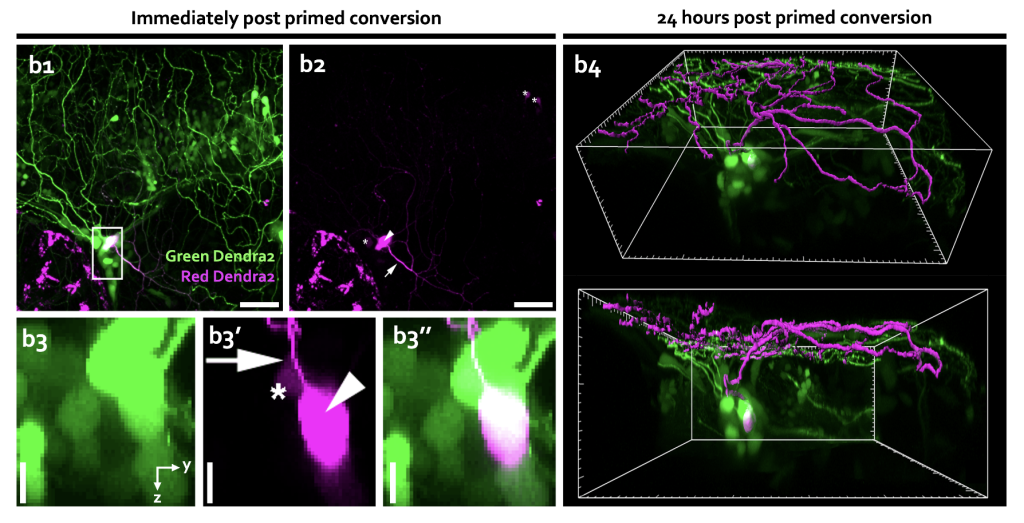
Outlook
In the last 6 years since primed conversion was introduced to the scientific community as a new photoconversion methodology, we have witnessed a great development in terms of understanding the nature of the process and even engineering proteins. However, primed conversion can be further improved, and research is still on-going. Until now, primed conversion has been only achieved using confocal laser scanning microscopy which is limited both in acquisition time and depth. In addition, current pr-FPs are less than 20 in total which limits their use and adaptation into already established experimental flows. Overall, I believe that primed conversion holds a great potential to understand the dynamics of complex biological systems and processes, such as embryo development and disease progression, with single cell resolution. Finally, I hope that by the end of this post you will be at least tempted to try primed conversion in your confocal microscopes, especially if you have already been working with photoconvertible FPs. And if not feel free to reach out, as we will be happy to assist.
Acknowledgements
I would like to wholeheartedly thank Periklis (Laki) Pantazis and Christina Vagena-Pantoula for their precious feedback on the post and Esperanza Agullo-Pascual for the more than kind support.
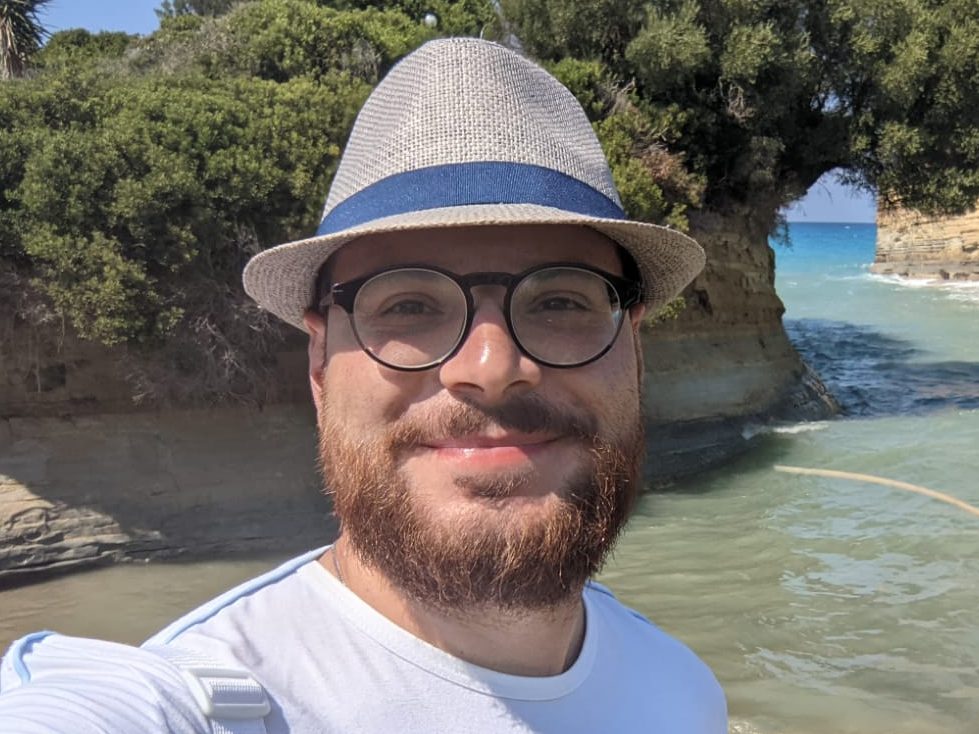
Konstantinos Kalyviotis, PhD Student
Laboratory of Advanced Optical Precision Imaging
Department of Bioengineering, Imperial College London, UK
References:
1. W. P. Dempsey et al., In vivo single-cell labeling by confined primed conversion. Nature methods 12, 645-648 (2015).
2. M. A. Mohr, P. Pantazis, Primed Conversion: The New Kid on the Block for Photoconversion. Chemistry (Weinheim an der Bergstrasse, Germany) 24, 8268-8274 (2018).
3. S. Yaganoglu et al., GenEPi: Piezo1-based fluorescent reporter for visualizing mechanical stimuli with high spatiotemporal resolution. bioRxiv, 702423 (2019).
4. A. Y. Sonay et al., Biodegradable Harmonophores for Targeted High-Resolution In Vivo Tumor Imaging. ACS Nano, (2021).
5. G. H. Patterson, J. Lippincott-Schwartz, A photoactivatable GFP for selective photolabeling of proteins and cells. Science (New York, N.Y.) 297, 1873-1877 (2002).
6. R. Ando, H. Mizuno, A. Miyawaki, Regulated fast nucleocytoplasmic shuttling observed by reversible protein highlighting. Science (New York, N.Y.) 306, 1370-1373 (2004).
7. N. G. Gurskaya et al., Engineering of a monomeric green-to-red photoactivatable fluorescent protein induced by blue light. Nature biotechnology 24, 461-465 (2006).
8. M. Welling et al., Primed Track, high-fidelity lineage tracing in mouse pre-implantation embryos using primed conversion of photoconvertible proteins. eLife 8, (2019).
9. M. Welling;, K. Kalyviotis;, P. Pantazis;, Primed Track: reliable volumetric single-cell tracking and lineage tracing of living specimen with dual-labeling approaches. Bio-protocol, (2020).
10. M. A. Mohr et al., Rational Engineering of Photoconvertible Fluorescent Proteins for Dual-Color Fluorescence Nanoscopy Enabled by a Triplet-State Mechanism of Primed Conversion. Angewandte Chemie (International ed. in English) 56, 11628-11633 (2017).
11. B. Turkowyd et al., A General Mechanism of Photoconversion of Green-to-Red Fluorescent Proteins Based on Blue and Infrared Light Reduces Phototoxicity in Live-Cell Single-Molecule Imaging. Angewandte Chemie (International ed. in English) 56, 11634-11639 (2017).
12. W. Zhang et al., Optogenetic control with a photocleavable protein, PhoCl. Nature methods 14, 391-394 (2017).
13. M. A. Mohr, P. Pantazis, Single neuron morphology in vivo with confined primed conversion. Methods in cell biology 133, 125-138 (2016).
14. K. Kalyviotis, H. Qin, P. Pantazis, in Behavioral and Neural Genetics of Zebrafish, R. T. Gerlai, Ed. (Academic Press, 2020), pp. 309-322.