Technology highlights – Fluorescence Lifetime Imaging (FLIM)
Posted by Johanna Bischof, on 25 November 2020
Interview with Prof. Dolf Weijers and Prof. Joris Sprakel from Wageningen University, The Netherlands
Tell us a little bit about who you are and about the people involved in the work we are talking about today.
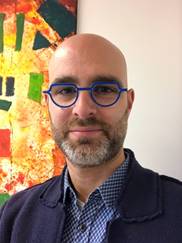
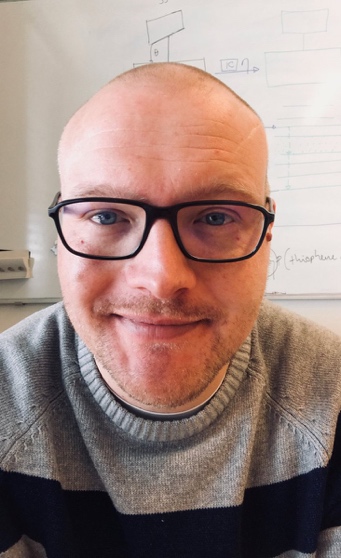
This work is a collaboration between the groups of Prof. Dolf Weijers and Prof. Joris Sprakel at Wageningen University, conducted by Lucile Michels (PhD student) and Vera Gorelova (postdoc). The chemistry group of Sprakel focusses on understanding how mechanical cues influence materials and biological systems, and a big part of this effort is geared to the discovery of new ways to visualise mechanical patterns with nanoscopic resolution. The Weijers team studies plant development, with emphasis on the cellular processes underlying polarity, oriented cell division and multicellularity. This project emerged at the interface of these groups, where the tools developed by Sprakel could be used by the team of Weijers to begin a deeper understanding of how mechanics can be instructive signals to guide plant development. Wageningen University, where we work, hosts the Advanced Light Microscopy and Molecular Imaging Node Wageningen of Euro-BioImaging.
We are today talking about Fluorescence Lifetime Imaging Microscopy (FLIM) and the tools you developed to use this technique to measure viscosity in plant cells. Can you please briefly tell us how FLIM works and what it can be used for?
Fluorescence Lifetime Imaging (FLIM) has become a powerful tool in cell biology as it allows combining the information on the excited state lifetime of fluorescent probes (with sub-nanosecond time resolution) with the spatial imaging resolution offered by confocal microscopy. This spatially-resolved spectroscopic imaging method enables contrast on the basis of emission intensity as well as on fluorescence lifetime. The method is readily amenable to working on living (non-fixed) cells and tissues, offers sub-cellular resolution (typically around 300-500 nm). In particular when combined with fluorescent reporters, probes whose fluorescence lifetime changes in a predictable manner under the action of some physical property of interest, FLIM enables us to construct functional images with a quantitative character. Very often, FLIM is also used in conjunction with FRET to provide structural measurements of protein conformations, or to probe biomolecular interactions, in-situ and at confocal imaging resolution.
Tell us a bit more about the project to measure microviscosity in plant cells that you did using FLIM. What scientific questions were you addressing?
There is an increasing body of evidence that mechanical signals, e.g. those emerging by the growth and division of cells on their neighbors, act as instructive biological signals that trigger biochemical processes. For example, the orientations of cell division, and thereby tissue geometry, are now known to be controlled in part by mechanical interactions between adjacent cells. Understanding how the laws of mechanics shape tissues and instruct a wide diversity of biological processes is one of the central aims in the emerging field of plant mechanobiology. However, it remained unclear how these mechanical patterns could be directly, and quantitatively measured, rather than deducing these on the basis of inference. This was the problem we addressed in this project: How can we map mechanical patterns in plant cells and tissues quantitatively, in-situ and with high resolution?
The use of these molecular reporters, in conjunction with quantitative FLIM, enabled us to construct the first micromechanical images of plant cells and tissues.
To resolve this methodological challenge, we combined FLIM imaging with a new class of fluorescent molecular sensors whose optical signature changes in response to changes in the mechanics of the material surrounding the probe. The molecules where chemically designed to target specific parts of the plant cell anatomy (cell wall, plasma membrane, cytosol and vacuole) to perform local mechanical measurements there. The use of these molecular reporters, in conjunction with quantitative FLIM, enabled us to construct the first micromechanical images of plant cells and tissues. You can read more about the work we did in this paper on Complete microviscosity maps of living plant cells and tissues with a toolbox of targeting mechanoprobes [1].
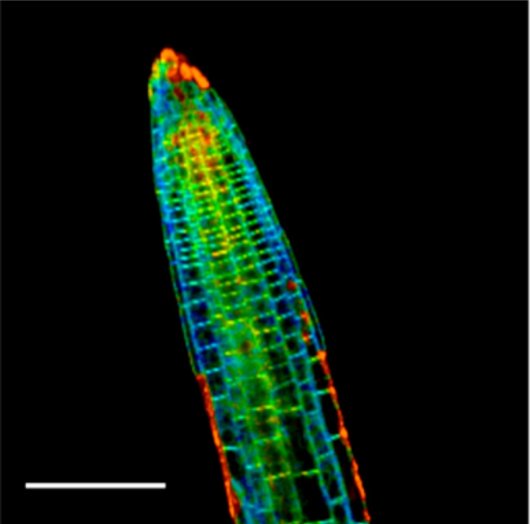
Our future work will be directed at employing the technique to answer new biological questions, such as what is the role of mechanical signalling in embryogenesis and what mechanisms do plant cells utilize to accommodate external mechanical stimulation. Moreover, we are exploring the use of this approach also in other organisms, such as plant pathogens that exploit mechanical stress to gain entry into their host and in which the visualization of the phenomena could bring new light to how pathogenic invasion is achieved.
Why is FLIM best suited to address this question?
These probes were specifically designed to work with FLIM, as this technique offers the possibility of doing highly quantitative measurements of the optical properties of a probe, much more so than ratiometric imaging approaches, while maintaining the spatial resolution and optical sectioning offered by confocal microscopy. Here FLIM is simply the best technique to leverage an accurate measurement with a high spatial resolving power.
It sounds like the probes that you developed to measure microviscosity in different part of the plant cell with FLIM were absolutely crucial for the success of the project. Can you tell us about how these probes work and how you developed them?
These fluorescent mechanosensors are based on a molecular rotor, a molecule composed of two distinct parts connected by a rotating carbon-carbon bond. When the molecule is brought to the excited state, it has the tendency to undergo this rotation, which opens a new decay pathway of the excited state by non-radiative means. The relative rate of intramolecular rotation versus fluorescence emission is probed by the fluorescence lifetime and depends sensitively on the ’fluidity’ of the surrounding medium, as the rotation involves hydrodynamic flows at the molecular scale. In this way, if the fluidity of the surrounding medium changes, the rate of the intramolecular rotation changes, and this can be detected by a change in the fluorescence lifetime. Through careful calibration, this opens the way to mechanical measurements at the molecular scale.
These molecular rotors have been known for at least 20 years, but mostly utilized in polymer science, and some studies in the membranes of mammalian cells. We set ourselves two challenges: make them suitable for use in plants and target not only membranes but also other essential parts of the plant cell anatomy involved in mechanical signalling. To this end, we started with a well-established basic molecular rotor, based on a BODIPY motif, and chemically modified it to spontaneously partition in the plant plasma membrane, cell wall, cytosol and vacuole. Combined with FLIM, this set of 4 mechano-optical probes enables the construction of complete microviscosity (or fluidity) maps of cells and tissues.
What are some challenges of using FLIM microscopy? What did you have to pay special attention to when performing these experiments?
In working with plants, one always needs to be cautious of autofluorescence through careful analysis of the underlying fluorescence lifetime data and the possible use of time-gating to eliminate autofluorescence signal. One drawback of FLIM imaging is that image acquisition can be somewhat slower than for conventional confocal imaging, such that the method as implemented is currently restricted to live-cell or live-tissue imaging in which dynamical processes occur on timescales slower than the acquisition rate (typically 30s – 1min per frame).
What other services do you provide in your facility that would be useful in combination with this type of microscopy?
Recently our Wageningen UR Microspectroscopy Centre, which is part of the Advanced Light Microscopy and Molecular Imaging Node Wageningen of Euro-BioImaging invested in a new instrument capable of rapid multiphoton 3D FLIM imaging, which opens the opportunity to look deeper inside opaque tissues and monitor faster processes. Multiphoton imaging will allow us to explore and visualize biology in regions that have remained hidden for conventional microscopy.
Want to use FLIM via the Euro-BioImaging service?
Euro-BioImaging is the European landmark research infrastructure for biological and biomedical imaging as recognised by the European Strategy Forum on Research Infrastructures (ESFRI). All scientists, regardless of their affiliation, area of expertise or field of activity can benefit from Euro-BioImaging’s pan-European open access services. By facilitating user access to high quality imaging facilities, resources, and services, with a constantly evolving technology offer, Euro-BioImaging will boost the productivity and impact of research across Europe.
Potential users of the Euro-BioImaging technologies are encouraged to submit project proposals via our website. To do so, you can LogIn to access our application platform, choose the technology you want to use and the facility you wish to visit, then submit your proposal. By using Euro-BioImaging, users will benefit from advice and guidance by technical experts working at the Nodes, training opportunities, and data management services.
For more information visit our website at www.eurobioimaging.eu or contact us at info@eurobioimaging.eu
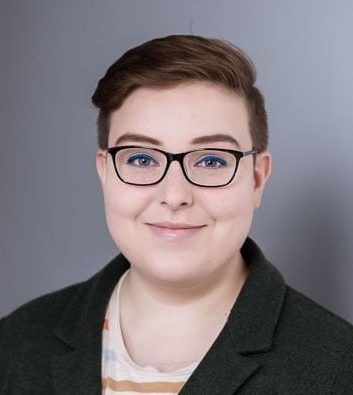
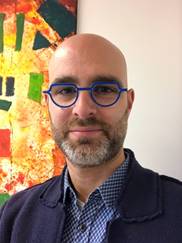
Dolf Weijers
Wageningen University
The Netherlands
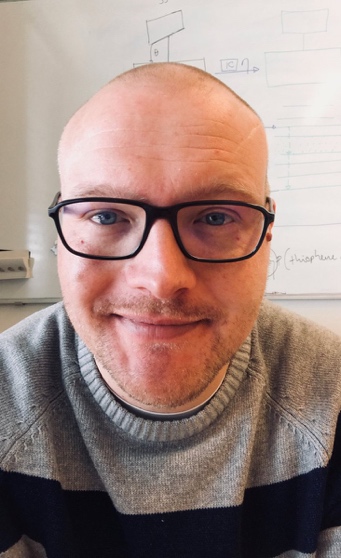
Joris Sprakel
Wageningen University
The Netherlands