Upcoming webinar: A Deep Dive into Biomolecular Condensates Using Single-Molecule FRET
Sponsored by PicoQuant, on 22 November 2023
Cells house organelles enclosed by membranes, which partition cellular components. Recent captivating research suggests an additional means of intracellular compartmentalization and organization, namely liquid-liquid phase separation of proteins and nucleic acids, forming noncanonical membrane-less organelles. These dynamic biomolecular condensates, resembling liquids, may undergo undesirable irreversible phase transitions, leading to gel-like or solid-like amyloid aggregates. Such transformations are linked to various severe human diseases.
In a brand-new paper, Ashish Joshi and colleagues from the team of Samrat Mukhopadhyay at IISER Mohali take a close look at the molecular events during phase separation of the prion-like domain of the FUS protein with single-molecule fluorescence methods.
Save the date: Webinar with Professor Samrat Mukhopadhyay
If you want to learn more about these techniques and what they revealed about the complex behavior of the FUS low-complexity domain during liquid-liquid phase separation, mark your calendar for our upcoming webinar:
Thursday January 11th 2024
2:00 pm London | 3:00 pm Berlin | 7:30 pm Mumbai
Online @ Zoom, registration via FocalPlane will open soon – stay tuned!
The webinar is co-hosted by FocalPlane and PicoQuant. To already find out more about PicoQuant`s newest fluorescence microscope Luminosa for single-molecule fluorescence studies, check our website:
Fused in Sarcoma (FUS), an archetypical phase-separating protein
Fused in Sarcoma (FUS) is a multifunctional RNA/DNA-binding protein. It is predominantly localized in the nucleus, but it can shuttle between the nucleus and the cytoplasm. FUS plays a crucial role in various cellular processes, including RNA processing, RNA transport, DNA damage repair, and the formation of stress granules. The N-terminal domain of FUS (FUS-LC) is an intrinsically disordered prion-like low-complexity domain, that can undergo liquid-liquid phase separation (LLPS). LLPS is increasingly recognized as a fundamental process in cellular organization and function. It is implicated in the formation of membrane-less organelles or biomolecular condensates, such as stress granules, which play a role in the dynamic regulation of cellular processes, including RNA metabolism. Intrinsically disordered proteins (IDPs) play crucial roles in LLPS. They can promote phase separation, regulate the dynamics of phase separation, serve as scaffolds of liquid droplets, and more.
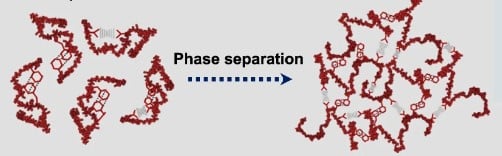
While FUS is essential for normal cellular function, mutations, or dysregulation of the FUS gene have been associated with certain neurodegenerative diseases, particularly amyotrophic lateral sclerosis (ALS) and frontotemporal lobar degeneration (FTLD). In these diseases, solid-like aggregates of FUS in the cytoplasm of neurons has been observed.
The question is: How does sequence-encoded conformational plasticity determine transient intermolecular interactions which lead to formation of liquid-like condensates? And which impact do pathological mutations have on the phase behavior?
Since LLPS is a multifaceted and context-dependent phenomenon, researchers use a combination of experimental and computational methods to gain a comprehensive understanding.
A toolkit of complementary single-molecule fluorescence methods to study FUS
One key instrument to study LLPS is the fluorescence microscope, which allows researchers to visualize the formation, dynamics and behavior of liquid droplets in real-time.
Simple observation under the microscope can already provide evidence for liquid-like states of round objects:
- Two droplets fuse upon touching, and together take on a spherical shape
- Droplets deform in shear flows
- Droplets rearrange components internally and exchange material with the surrounding medium
Fluorescence recovery after photobleaching (FRAP)
When applying FRAP to study LLPS, researchers photobleach a small region within the phase-separated condensate. The recovery of fluorescence over time in the bleached region can provide information about the mobility, viscosity, and molecular interactions of molecules within the dense phase.
Rapid and complete FRAP of droplets of FUS-LC proves their liquid properties.
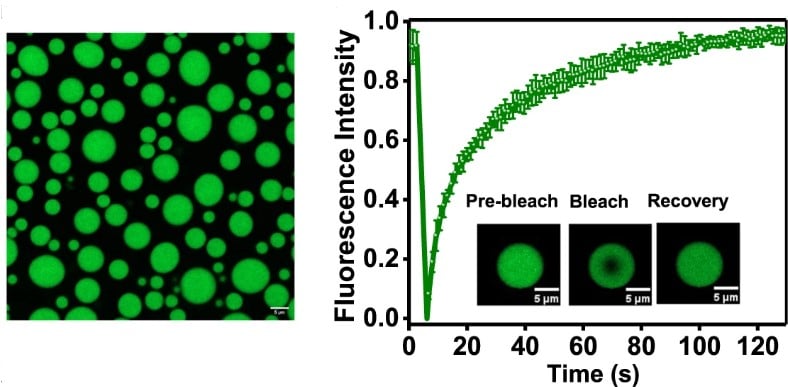
Distance estimation from single-molecule FRET reveals coexisting structural subpopulations
Förster Resonance Energy Transfer, short FRET, is a physical phenomenon where energy from an excited donor fluorophore is transferred to a nearby acceptor fluorophore, which subsequently emits a fluorescence photon. The transfer efficiency depends on the distance between the fluorophores, which is in the range of 1 to 10 nm. Therefore, FRET is used for measuring nm distances, distance changes, and detecting molecular interactions. Single molecule FRET (smFRET) is applied to individual molecules rather than a bulk sample, and thus
- Observes distributions within a population
- Visualizes conformational subpopulations
- Reports state transition dynamics
When applied to FUS, smFRET showed two coexisting structural subpopulations in the monomeric form, as well as a structural expansion and an increase in conformational heterogeneity upon phase separation.
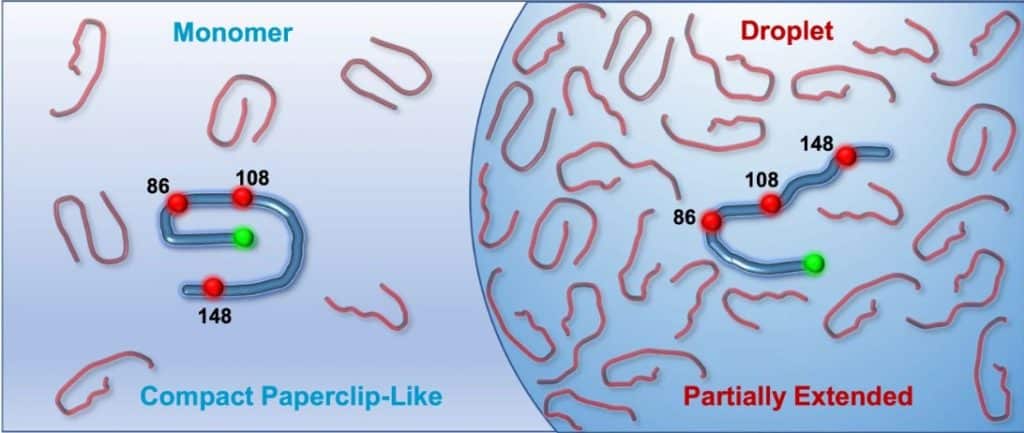
Translational diffusion times obtained from Fluorescence correlation spectroscopy (FCS)
FCS provides both the local concentration of the labeled molecules and their diffusion characteristics. Droplets form when the concentration of the involved components exceeds a certain threshold. That is why measuring local concentrations with FCS is one fundamental method for studying phase separation.
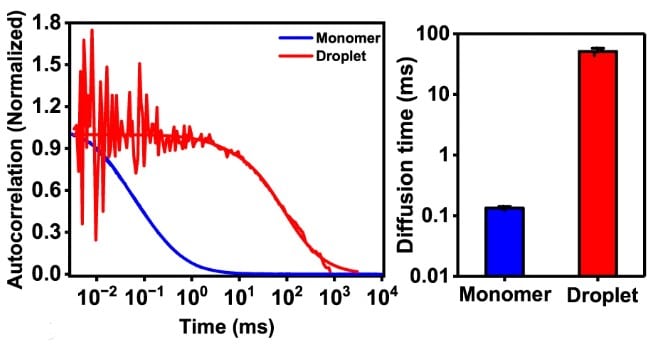
By comparing the translational diffusion speeds in the dense and dilute phase one can detect increased viscosity inside droplets. The diffusion time of FUS-LC increases 400 times, indicating a highly crowded and viscous environment inside the droplets.
Rotational dynamics observed with anisotropy
While FCS reports on translational diffusion, fluorescence anisotropy informs about rotational diffusion and reorientation dynamics.
Steady-state anisotropy
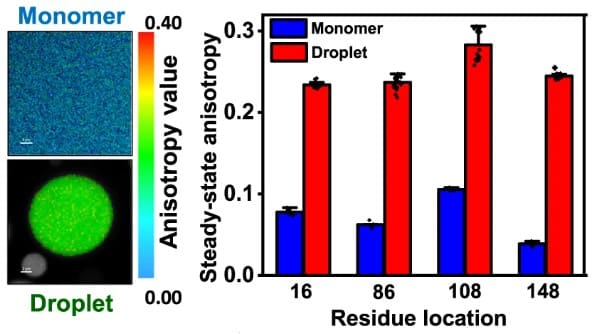
With steady-state anisotropy imaging one obtains the average local rotational dynamics of the labeled molecules. Comparing steady-state anisotropy values of dense and dilute phase can indicate for example dampening of the rotational motion due to higher viscosity inside droplets, as is the case for FUS.
Time-resolved anisotropy
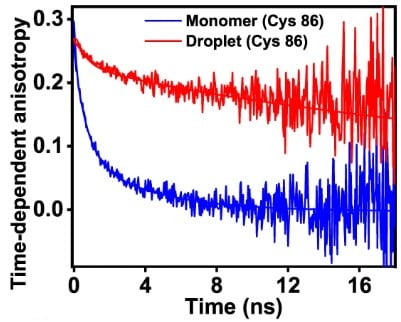
From time-resolved anisotropy decays, one can obtain rotational correlation times via multiexponential fitting. These correspond for example to rotational diffusion speed of folded proteins, or to reorientation dynamics of the disordered protein chain as in the case of FUS.
Comparing the rotational correlation times obtained in dilute and dense phase yields an increase in chain reorientation. This corresponds well to the increased steady-state anisotropy in FUS condensates.
Joshi, A., Walimbe, A., Avni, A. et al. Single-molecule FRET unmasks structural subpopulations and crucial molecular events during FUS low-complexity domain phase separation. Nat Commun 14, 7331 (2023). https://doi.org/10.1038/s41467-023-43225-y
* All figures adapted from Joshi, A. et al. under the Creative Commons Attribution 4.0 International License: http://creativecommons.org/licenses/by/4.0/