What Happens When Light Meets Cell: Is It Stress, Signal, or Something More?
Posted by Neelabh Datta, on 30 December 2024
In the hub of cellular responses, how does a beam of light trigger a cascade of molecular changes in plant cells? This question solves the mystery of photostimulation-induced stress and signaling, especially at the critical contact points between the endoplasmic reticulum (ER) and chloroplasts. These dynamic cellular sites act as points for communication, resource exchange, and, intriguingly, stress responses to environmental stimuli. But is the observed transient ER stress merely a result of photostimulation, or does it unveil a deeper interaction between light, cellular structures, and molecular signaling?
To address this, researchers from Texas A&M University conducted a series of controlled photostimulation experiments, revealing that specific wavelengths of light, notably 405 nm, elicit a robust calcium wave across plant cells. Interestingly, light of similar power at 488 nm caused only minor alterations in chloroplast position without producing a calcium wave. This disparity suggests a unique photostimulation mechanism at play, with 405 nm light having distinct effects not replicated by other wavelengths.
Calcium waves in plant cells are often associated with stress responses, including wounding or osmotic shock. The possibility that photostimulation-induced calcium waves mimic wounding effects was explored previously. Those studies showed that wounding generates calcium waves mediated by plasma membrane glutamate receptor-like channels, which are sensitive to the metal lanthanum. However, the calcium waves triggered by 405 nm photostimulation displayed no sensitivity to lanthanum, suggesting a different origin. Instead of external influx through plasma membrane channels, the calcium surge appears to originate from internal organelles.
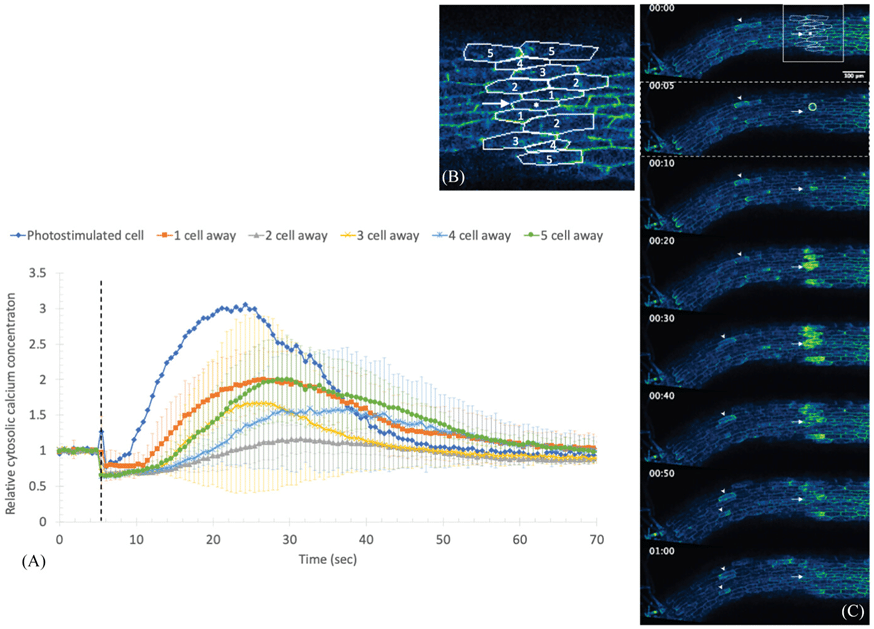
Illustration of the spatiotemporal dynamics of calcium signaling in plant cells upon photostimulation. (A) The graph displays relative cytosolic calcium concentration changes over time, highlighting the rapid peak in the photostimulated cell (blue) followed by delayed and attenuated responses in adjacent cells up to five cells away. (B) The labeled micrograph depicts the cellular arrangement, where the photostimulated cell and neighboring cells (numbered 1-5) are tracked for calcium signaling propagation. (C) The time-lapse images show fluorescence intensities corresponding to calcium concentration in the cytosol, with brighter areas indicating higher calcium levels. Together, they reveal the wave-like propagation of calcium signaling from the site of photostimulation, which diminishes in magnitude as the signal travels further from the source. Image adapted from https://doi.org/10.1111/jmi.13377
This finding shifts focus to the ER, which displayed an accompanying pH change during the calcium wave. Using the D4ER cameleon sensor, researchers initially suspected that the ER might release calcium into the cytoplasm. However, further analysis revealed no significant decrease in ER calcium levels, raising doubts about this hypothesis. The pHluorin-ER and YFP-HDEL probes, both sensitive to pH changes, indicated an ER lumen pH shift rather than calcium release. These results prove the complexity of dissecting calcium signaling, particularly when probe sensitivities may confound interpretations.
Reactive oxygen species (ROS) emerged as another potential participant in this signaling network. ROS are well-known for activating plasma membrane calcium channels during stress responses, such as osmotic shock or wounding. This study’s dichlorofluorescein diacetate (DCFDA) staining revealed ROS waves following photostimulation, particularly in the nucleus, mitochondria, and chloroplasts. The ROS increase was observed almost simultaneously across the cell, independent of the slower calcium wave propagation.
This rapid ROS response raises fascinating questions. Is the ROS wave driven by the ER’s pH change, cytoplasmic calcium fluctuations, or another unidentified signal? The absence of ROS changes in the cytosol and cell wall supports the idea that the ROS signal might originate from organelle-specific interactions, possibly mediated by ER contact sites with mitochondria and chloroplasts. These open avenues for exploring ER-specific ROS dynamics using redox-sensitive probes targeted to various organelles.
The ER-chloroplast contact site stands out as a focal point in this study, where photostimulation appears to trigger localized stress responses. These contact sites, known for their role in resource exchange and signaling, likely facilitate the observed calcium and ROS waves. However, the nature of the transmitted signal remains elusive. While the ER stress and calcium wave are confined to the photostimulated cell, the calcium wave’s transmission to adjacent cells hints at an alternative mechanism, possibly involving plasmodesmata.
Interestingly, the photostimulation response is not merely a product of chlorophyll excitation. Experiments using longer wavelengths (488 nm and 640 nm) showed no calcium wave, despite their overlap with chlorophyll’s absorption peaks. Instead, the sensitivity to 405 nm and UV light (380 nm) points to the involvement of specific photoreceptors. Cryptochrome 3, a poorly understood receptor localized in chloroplasts and sensitive to near-UV light, emerges as a strong candidate. Mutant studies and action spectrum analyses are underway to confirm its role. Advanced microscopy techniques like confocal microscopy allowed precise photostimulation and real-time imaging of calcium waves and ROS dynamics. By targeting specific cellular regions with 405 nm laser light, researchers induced localized responses while minimizing potential artifacts. High-resolution imaging captured the rapid spread of calcium waves and ROS signals, providing valuable insights into their temporal and spatial dynamics.
For instance, pH-sensitive fluorescent probes like pHluorin-ER and YFP-HDEL illuminated the ER’s pH changes during the calcium wave. Similarly, DCFDA staining highlighted ROS accumulation in specific organelles, revealing their distinct response patterns. The unique response to 405 nm light raises questions about its physiological relevance. Could this wavelength mimic natural environmental cues, such as short UV exposures in sunlight? The study’s findings suggest that low-power UV light can induce similar calcium waves, hinting at a broader ecological function. Further experiments with cryptochrome 3 mutants and extended action spectrum analyses could provide answers on the evolutionary significance of this response. Phototropins, well-known photoreceptors involved in chloroplast movement, were ruled out due to their insensitivity to 405 nm light. This highlights the specificity of the observed response and directs attention toward lesser-known photoreceptors like cryptochrome 3. Understanding how these receptors integrate light signals with cellular stress responses could reveal novel adaptive mechanisms in plants.
As the pathways of light-induced signaling in plant cells are revealed, one question lingers: How do these responses integrate with broader cellular networks to influence plant behavior and adaptation? The answers may hold the key to unlocking new strategies for enhancing plant resilience in changing environments.
Original Article
Maynard, S. N., & Griffing, L. R. (2024). The photosensitive endoplasmic reticulum-chloroplast contact site. Journal of Microscopy, 1–16. https://doi.org/10.1111/jmi.13377